冰川底部富铁溶液氧化沉淀: 塔里木北缘新元古界库鲁克赛铁矿床的成因*
【类型】期刊
【作者】高丙飞,吴昌志,李伟强,杨涛,叶辉,雷如雄,刘倩(南京大学地球科学与工程学院;江苏省地质调查研究院基础地质研究所;长安大学地球科学与资源学院)
【作者单位】南京大学地球科学与工程学院;江苏省地质调查研究院基础地质研究所;长安大学地球科学与资源学院
【刊名】矿床地质
【关键词】 地球化学;新元古代;铁建造;冰川作用;库鲁克赛;塔里木
【资助项】国家自然科学基金(编号:U1603114);中国地质调查局工作项目(编号:1212011140056)联合资助
【ISSN号】0258-7106
【页码】P1-26
【年份】2019
【期号】第1期
【期刊卷】1;|7;|8;|4;|5;|2
【摘要】新元古代沉积变质铁矿床是继大氧化事件(GOE)后,沉积间断10亿年(~1800 Ma至~750 Ma)之后,再次大规模出现的一种沉积铁建造类型。这类铁建造与新元古代冰碛岩密切伴生,是新元古代雪球地球事件的重要证据。文章选择与新元古代雪球地球事件有关的沉积变质赤铁矿床—库鲁克赛铁矿进行研究,通过锆石U-Pb定年和区域地层对比工作限定其形成时代为新元古代青白口纪末期—南华纪早期。锆石年龄谱值对比和岩相学研究表明,铁矿床中的碎屑物质主要来自于青白口系下部独断山组石英砂岩地层。主、微量元素研究表明,库鲁克赛铁矿形成于相对富氧或者从贫氧向富氧变化的环境,其成矿元素应主要与陆源物质风化有关,可能有少量成矿元素来自于低温海底热液或海水。笔者认为,库鲁克赛铁矿的形成与成冰纪冰水沉积作用有关,来自冰下水体、从冰下通道中流出的富铁缺氧水溶液与富氧的表层海水混合时,成矿元素快速氧化沉淀,胶结冰水中的近源砾石,进而形成了此种富铁砾岩型铁矿。
【全文】 文献传递
冰川底部富铁溶液氧化沉淀: 塔里木北缘新元古界库鲁克赛铁矿床的成因*
摘 要 新元古代沉积变质铁矿床是继大氧化事件(GOE)后,沉积间断10亿年(~1800 Ma至~750 Ma)之后,再次大规模出现的一种沉积铁建造类型。这类铁建造与新元古代冰碛岩密切伴生,是新元古代雪球地球事件的重要证据。文章选择与新元古代雪球地球事件有关的沉积变质赤铁矿床—库鲁克赛铁矿进行研究,通过锆石U_Pb定年和区域地层对比工作限定其形成时代为新元古代青白口纪末期—南华纪早期。锆石年龄谱值对比和岩相学研究表明,铁矿床中的碎屑物质主要来自于青白口系下部独断山组石英砂岩地层。主、微量元素研究表明,库鲁克赛铁矿形成于相对富氧或者从贫氧向富氧变化的环境,其成矿元素应主要与陆源物质风化有关,可能有少量成矿元素来自于低温海底热液或海水。笔者认为,库鲁克赛铁矿的形成与成冰纪冰水沉积作用有关,来自冰下水体、从冰下通道中流出的富铁缺氧水溶液与富氧的表层海水混合时,成矿元素快速氧化沉淀,胶结冰水中的近源砾石,进而形成了此种富铁砾岩型铁矿。
关键词 地球化学;新元古代;铁建造;冰川作用;库鲁克赛;塔里木
沉积变质型铁矿是中国十分重要的铁矿类型(李志红等, 2008; 李厚民, 2012; 李延河等, 2014), 主要形成于前寒武纪,大规模产出于太古代—古元古代(Goodwin, 1973; Gross, 1980; 1983; Ilyin, 2009; 李延河等, 2012; 刘清泉等, 2014),通常由硅质条带和铁质条带构成,两者互层交替产出,也被称为条带状铁建造(BIF)。作为前寒武纪特有的地质产物和早期地壳的重要组成部分,沉积变质铁矿床不仅具有重要的经济价值,还记录了早期地球的演化信息,是研究前寒武纪大气和海洋的重要线索(Cloud, 1973; Holland, 1973; James, 1983; Walker, 1984; Derry et al., 1990; Huston et al., 2004; 王长乐等, 2012; 李志红等, 2014)。
新元古代条带状铁矿床是继大氧化事件(GOE)后,间断10亿年左右(~1800 Ma至~750 Ma)又再次大规模出现的一种沉积变质铁矿类型(Hoffman et al., 1998; Bekker et al., 2010; 李厚民,2012; Baldwin et al., 2012)。与太古代—古元古代条带状铁建造不同,这类铁矿床常具有粒状构造,多不具有明显的条带,因此也被称为GIF(Granular Iron Formation)(Klein et al., 1993; Manikyamba, 1999; Trendall, 2002; Klein, 2005; 王长乐等, 2012; Angerer et al., 2016)。虽然新元古界铁矿床的规模和经济价值远小于太古代的阿尔戈马型和古元古代的苏必利尔型沉积变质铁矿,但其分布却更为广泛,在北美、南美,非洲、澳大利亚、西伯利亚、中亚、印度及华南扬子地块等均有报道(图1;Sims et al., 1984; 汤家富等,1987; Lottermoser et al., 2000; Young, 2002; 沈保丰等,2005; Carlos et al., 2007; Halverson et al., 2011)。
新元古代条带状铁建造的形成时间从780 Ma一直持续到630 Ma。新元古代氧化事件(NOE)通常被认为是其形成的原因之一(Spence et al., 2016; Och et al., 2012; Hayes et al., 2006)。前期海洋缺氧环境的产生,矿床学家将其归结为雪球地球事件(Klein et al., 1993; Hoffman et al., 1998; 2002)。正是由于冰川的覆盖作用,导致冰下水体具有了贫氧、富铁和高盐的特征;间冰期时,由于冰川融化,进而导致富铁水体氧化而形成相应的铁矿床。实际上,新元古代铁矿并不像太古代—古元古代铁矿床那样(以磁铁矿、赤铁矿或其他矿物相为主),其成矿矿物组成往往是排外性的赤铁矿(Klein et al., 1993; Cox et al., 2013),常与冰碛岩相伴生,反映出新元古代中期极端的气候环境(Cox et al., 2013)。Canfield等(1996)指出,在1000~640 Ma之间,地球外部大气的氧含量就已经逐渐升高至现代氧含量水平的5%~18%。在马雷诺冰期之后,深海开始被氧化,铁物质再次大量沉淀(Fike et al., 2007; Canfield et al., 2007)。
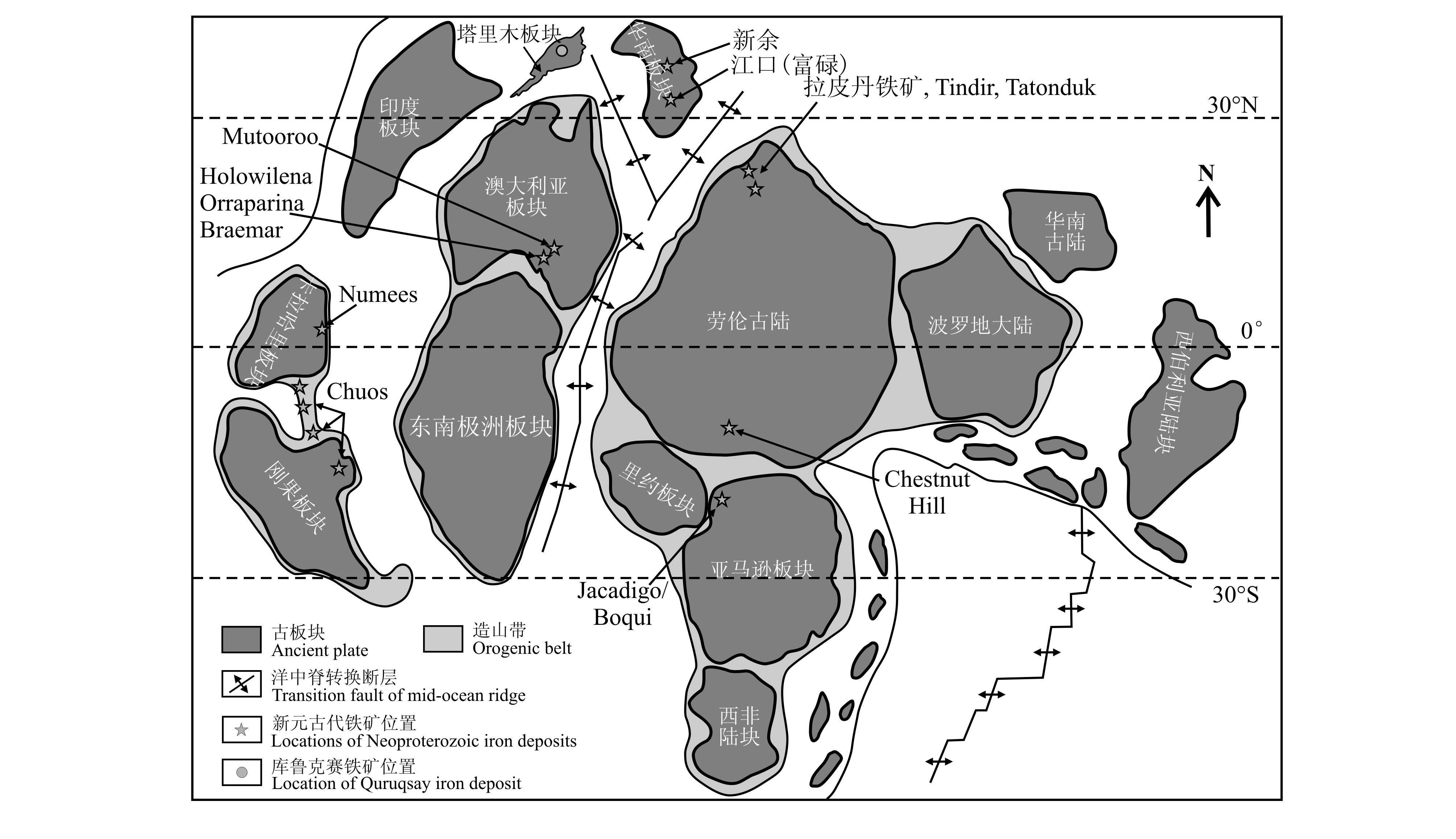
图 1 Rodinia超大陆中的新元古界铁建造分布示意图(据Torsvik, 2003; Ilyin, 2009; Li et al., 2013; Cox et al.,2013修编)
Fig. 1 Sketch map of Neoproterozoic iron formations in the Rodinia Supercontinent (modified after Torsvik, 2003; Ilyin, 2009; Li et al., 2013; Cox et al., 2013)
塔里木克拉通是中国3大古老克拉通之一,其北缘的库鲁克塔格地块前寒武系出露最为完整,并且普遍发育有含铁建造层。然而,由于环境恶劣、交通不便,前人对该地区新元古界铁建造尚未开展深入研究,对产于此区域的新元古代铁矿所具有的重要意义缺乏深入讨论。本文以塔里木北缘库鲁克塔格地块的库鲁克赛铁矿作为研究对象,通过详细的野外观察、相关地层的锆石年代学以及矿床地球化学工作,阐明该期铁建造的发育特征和形成时代,探讨塔里木新元古界铁建造形成过程、沉积环境和形成机制。
2 区域地质背景
库鲁克塔格地块位于塔里木盆地东北缘,总体近东西向伸展,是塔里木克拉通的重要组成部分。该地块北以辛格尔_铁门关断裂与南天山为界,南以孔雀河断裂与塔里木盆地早古生界接触(图2a、b;Shu et al., 2011)。该地块前寒武纪地层出露最为完整,可以划分为前寒武系变质基底和南华系—震旦系沉积盖层2个部分,呈现出典型的双层结构(冯本智等,1995)。
太古界由斜长角闪岩、灰色片麻岩、云母石英片岩夹少量不稳定大理岩透镜体及肉红色片麻状花岗岩等组成(陆松年, 1992;曹晓峰等, 2012),主要出露于库鲁克塔格地块的中部地区。
古_中元古界包括达格拉格布拉克群、兴地塔格群和爱尔基干群。达格拉格布拉克群主要由一套片麻岩和混合岩组成,其原岩形成于24亿年左右,后于18.5亿年发生强烈的变质作用。兴地塔格群和爱尔基干群主要是由云母片岩、片麻岩、闪长岩、大理岩以及由碎屑岩和碳酸盐岩组成的海相沉积序列(高振家, 1993; 胡霭琴等, 1997; Wu et al., 2014)。
新元古界沉积地层主要为青白口系帕尔岗塔格群和南华系—震旦系库鲁克塔格群。帕尔岗塔格群主要分布在东库鲁克塔格南坡帕尔岗塔格及库鲁克塔格、兴地塔格、雅尔当山等地。据新疆地质矿产局1∶50 000矿产地质调查地层划分方案,帕尔岗塔格群下部(独断山组)为一套厚度不大的变质粉砂岩、粉砂质页岩、石英岩地层,与蓟县系爱尔基干群呈假整合或整合接触;其上部(横断山组)为一套富含叠层石礁的厚层白云质灰岩、结晶灰岩、白云岩地层,与上覆库鲁克塔格群呈明显的不整合接触关系。高振家等(1984)将库鲁克塔格群自上而下划分为:震旦系汉格尔乔克组、水泉组、育肯沟组和扎摩克提组;南华系特瑞艾肯组、阿勒通沟组、照壁山组和贝义西组。
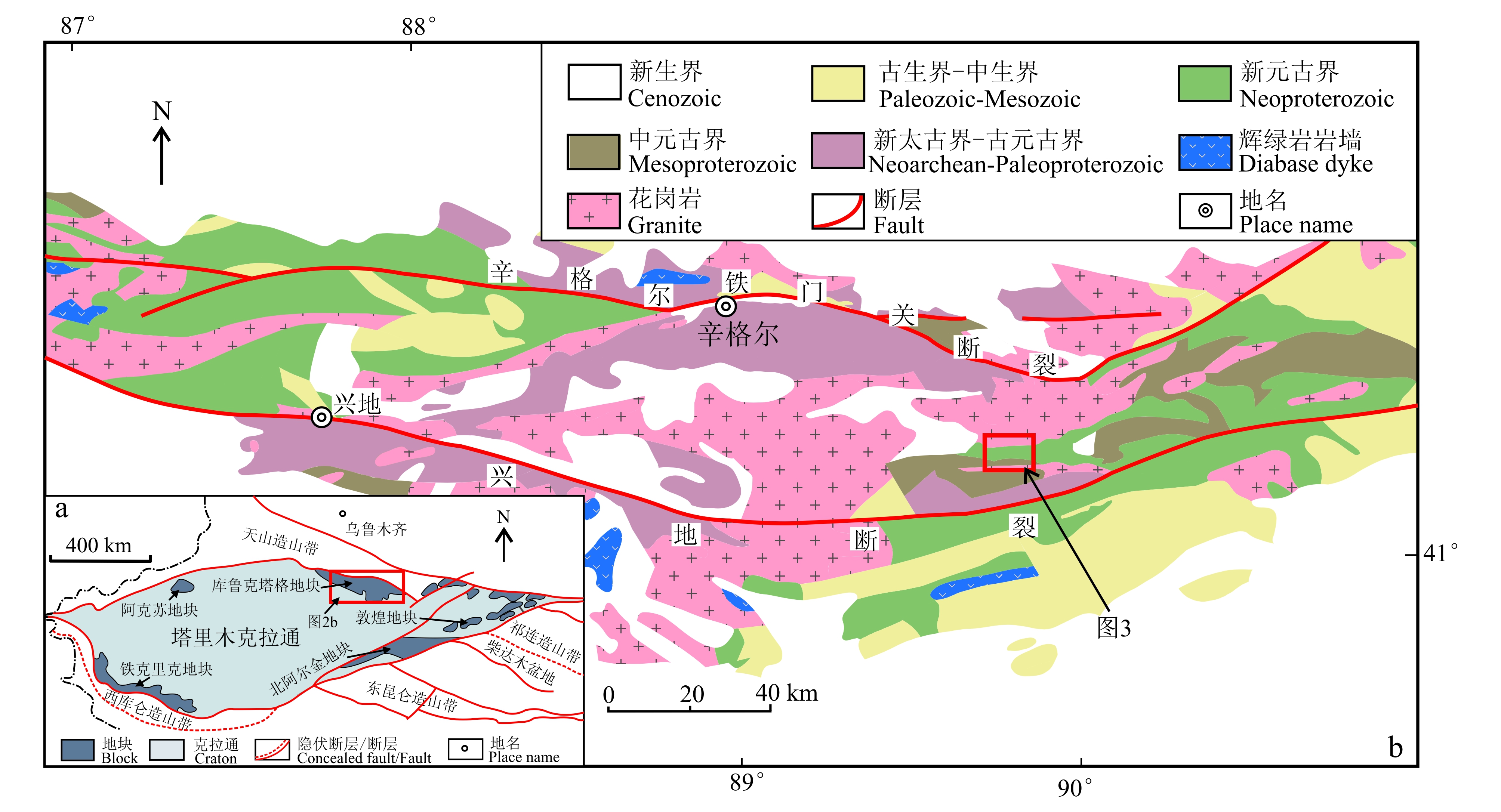
图 2 塔里木克拉通大地构造简图(a)和库鲁克塔格地块区域地质简图(b,据Wu et al., 2014修编)
Fig. 2 Location of the Tarim Craton (a) and regional geological map of the Quluketage block, northern Tarim Craton (b, modified after Wu et al., 2014)
3 矿区地质
库鲁克赛铁矿位于库鲁克塔格地块东段(图3)。矿区内出露的地层有新太古代喀拉阔雄岩群、古元古界兴地塔格群、中元古界长城系杨吉布拉克群和蓟县系爱尔基干群,新元古界青白口系帕尔岗塔格群和南华系—震旦系库鲁克塔格群(图3)。
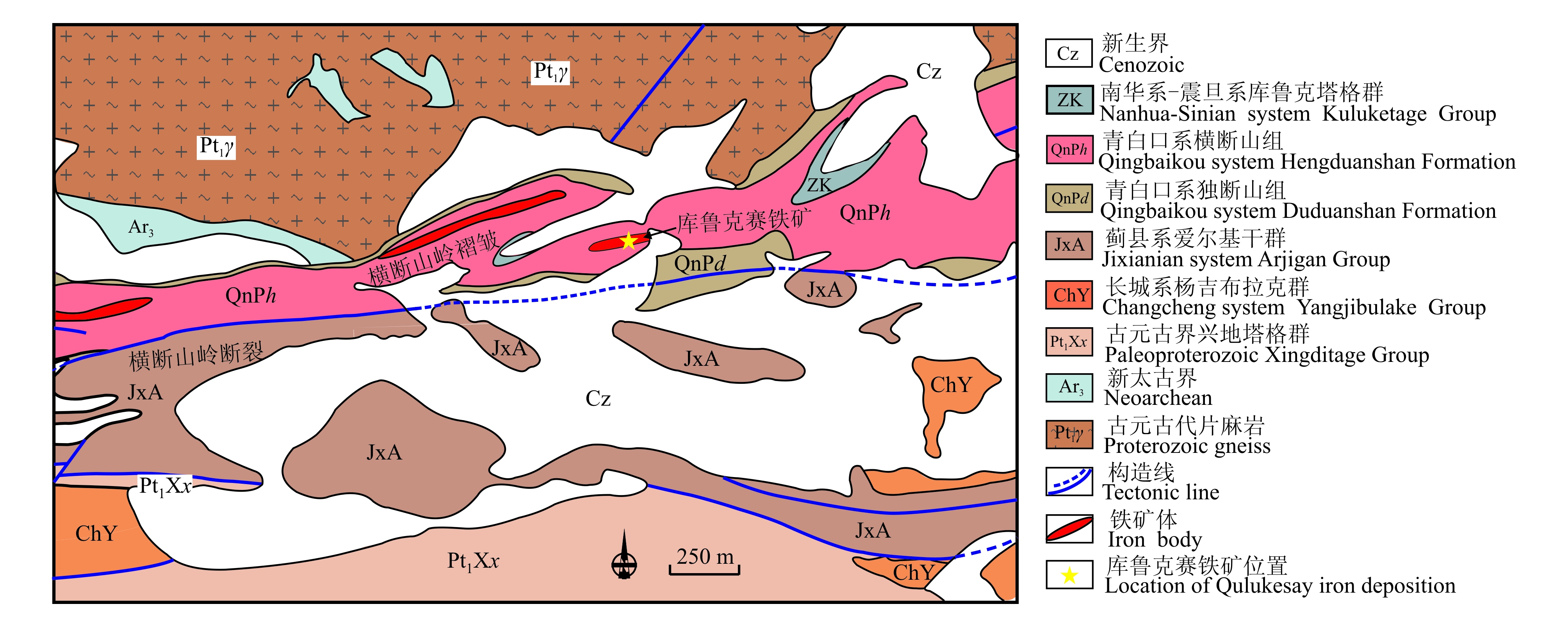
图 3 库鲁克赛铁矿区域地质简图(据新疆地质矿产局,1995修编)
Fig. 3 Regional geological map of the Quruqsay iron formation (modified after Xinjiang Bureau of Geology and Mineral Resources, 1995)
兴地塔格群、杨吉布拉克群和爱尔基干群主要出露矿区南部。兴地塔格群岩性为二云母石英片岩、石英片岩、大理岩。杨吉布拉克群底部为砾岩,向上变为石英岩、石英片岩,千枚岩。爱尔基干群为一套薄层状白云石大理岩,微晶不纯大理岩地层。
新元古界青白口系帕尔岗塔格群主要出露在矿区中部,呈狭长型,东西向延伸。自下而上可以细分为独断山组和横断山组。独断山组为一套厚度不大的变质石英砂岩夹长石石英砂岩地层,不整合覆盖于爱尔基干群之上。横断山组岩性为块状白云石大理岩、不纯微晶大理岩,与上覆的南华系—震旦系库鲁克塔格群呈角度不整合接触关系。库鲁克赛铁矿的矿体多呈透镜状,周围被横断山组白云岩地层包围,少数呈似层状角度不整合于横断山组之上。
南华系—震旦系库鲁克塔格群主要出露于矿区北部,横断山岭中部位置。岩性为紫红色砂岩、粉砂岩夹少量白云岩。
区内出露的褶皱为横断山岭褶皱,轴迹近东西向延伸,其西部(独断山一带)被震旦系库鲁克塔格群角度不整合覆盖,南侧被横断山断裂限制。褶皱总体为一向斜构造,核部与南翼往往因断层破坏而不完整。该褶皱平面上呈线性褶皱,轴面略有弯曲,总体走向75°,北翼产状近170°∠50°,南翼产状近350°∠65°(新疆地质矿产局, 1995)。
矿区内断裂发育,可见北东向和东西向2组断裂,性质皆为高角度逆断层(图4)。北东向断裂形成较晚,局部地段切割了铁矿体。铁矿体附近发现有一条辉绿岩岩脉,脉体呈北西向延伸,斜切铁矿体。
4 矿床特征
库鲁克赛铁矿区东西长约1500 m,南北宽约100~150 m。矿区内由东向西大体圈定出3个矿化地段,15个铁矿矿体。矿体一般沿地层走向断续出露,总体产状为350°∠60°,形态以似层状、透镜状为主。其中,规模大者长达295 m、宽50 m,小者仅长25 m,宽5 m,整体品位在30%~35%之间。
本文研究对象为第一个矿化段内的1号铁矿体。该矿体长约150 m,富矿体宽约6~15 m,走向近东西,倾角50°~70°。矿体中部被断层和韧性剪切带一分为二(图5a),韧性剪切带附近发生强烈的千糜岩化,并伴生断层角砾岩和碎裂岩(图5b)。
下部矿体(图5c)与横断山组大理岩呈角度不整合接触,受动力变质作用影响较小,岩性为富铁质砾岩(图5d)。上部矿体与上覆地层呈断层接触,整体岩性为含铁砾岩,矿体中的砾石含量很高,成分相对复杂。受动力变质作用影响,砾石常具压扁拉长形态,部分矿石中可见似坠石特征的石英砂岩岩屑(图5e),镜下可见有拖尾现象(图5f)。
矿体中矿石矿物主要为赤铁矿和少量镜铁矿,以胶结物的形式产于矿石之中(图5g)。下部矿体的脉石矿物含量较少,类型相对简单,主要为石英砂岩岩屑、碧玉、燧石等,粒径大小不一,大者可达15 cm×5 cm, 小者仅2 mm×1 mm。上部矿体受断裂构造的影响,脉石含量普遍较高,类型相对复杂,但仍以单颗粒石英、燧石和石英岩岩屑为主,大块砾石较多,大者可达30 cm×12 cm,一般3 cm×2 cm。
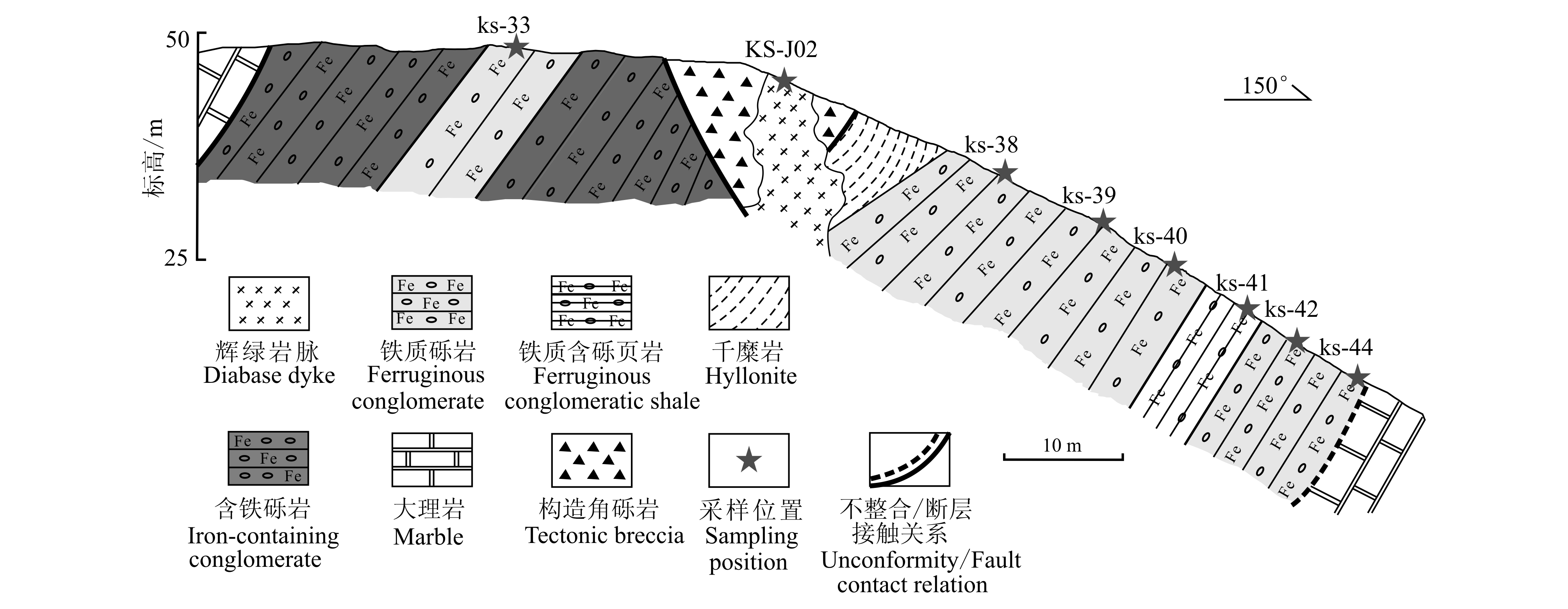
图 4 库鲁克赛铁矿地层剖面图及具体采样位置
Fig. 4 Geological section of the Quruqsay iron formation showing sampling sites
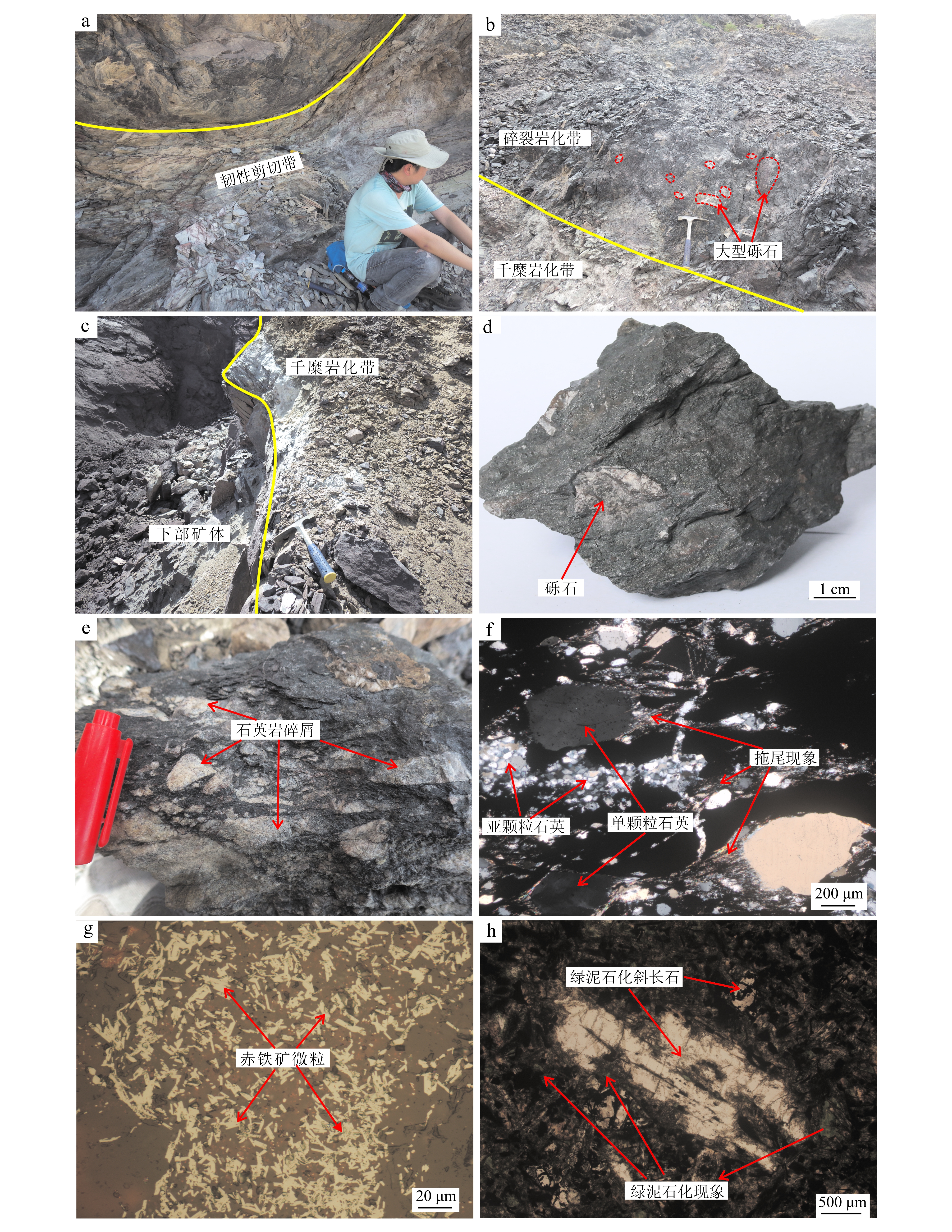
图 5 库鲁克赛铁矿野外(a~c)、手标本(d~e)及镜下(f~h)照片
a. 矿体中部韧性剪切带; b. 千枚岩与碎裂岩接触带,接触带附近的碎裂岩中见有较多大块砾石; c. 下部富铁矿体与千枚岩化带清楚的接触界面; d. 下部矿体中的富铁矿石; e. 样品ks_40中的似坠石现象; f. 样品ks_40中石英边部的拖尾现象(正交偏光); g. 样品ks_33中晶形较好的赤铁矿微粒(反射光); h. 样品KS_J02辉绿岩中的绿泥石化现象(单偏光)
Fig. 5 Field (a~c), hand specimens (d~e) and microphotographs (f~h) of the Quruqsay iron formation
a. Ductile shearing zone in the middle of the ore deposit; b. Contact zone of cataclasite and phyllonite in upper host rock of the deposit. The cataclasite strata contain abundant gravels; c. Clear contact interface between iron orebody and phyllic zone; d. The iron_rich ore in the lower orebody; e. Possible dropstone in the lower orebody (ks_40); f. Tailing feature of quartz grains in the ore (ks_40; crossed nicols); g. Euhedral platy hematite grains in the ironstone of the deposit (ks_33; reflected light); h. Chlorite alteration of diabase that intruded into the iron formation (KS_J02; plainlight)
矿体中部,韧性剪切带附近的辉绿岩岩脉风化蚀变较为强烈,镜下呈现出的较强的绿泥石化、泥化现象(图5h)。
5 分析方法与结果
5.1 锆石U_Pb定年
本次工作共采集3块样品开展锆石U_Pb定年分析工作,分别编号ks_1、ks_46、KS_J02。样品ks_1是采自铁矿体的铁矿石,质量约3 kg,岩性为富铁质砾岩。样品ks_46是采自矿体下伏的帕尔岗塔格群中下部的石英砂岩(北纬41°11′3.97″;东经89°47′56.83″),质量约2 kg,岩性为红褐色细粒石英砂岩。样品KS_J02是采自切穿铁矿体的的辉绿岩岩脉的辉绿岩,质量约5 kg。样品ks_46、KS_J02送至河北廊坊进行制靶和CL图像的拍摄工作。样品ks_1粉碎至50~100目,用常规浮选和磁选方法分选后,得到锆石近500粒,然后进行显微照相和制靶,显微照相和制靶流程参见文献(宋彪等, 2002)。
锆石测点位置的选取主要依据反射光、透射光照片和CL图像,力求避开裂隙和包裹体。LA_ICP_MS锆石微区U_Pb年龄测定工作在南京大学内生金属矿床国家重点实验室进行。使用了Agilent 7500a型MC-ICP_MS和Newwave UP 213激光剥蚀系统。详细的分析计算过程及精度控制见文献所述(Griffin et al., 2004; Jackson et al., 2004)。所得结果按照Andersen(2002)所述方法进行普通铅校正后,再由程序Isoplot3.23绘制U_Pb年龄谐和线图和年龄谱图(Ludwig, 2003)。在讨论中,年龄小于1000 Ma的锆石采用206Pb/238U年龄;年龄大于1000 Ma的锆石则采用207Pb/206Pb年龄。不和谐度大于5%或普通铅校正大于2%的数据将不参与讨论。
来自铁质砾岩层的样品(ks_1)共测试分析了90个点(表1),其中协和度>95%的测点87个(图6a)。由上述图表可知,锆石U_Pb年龄变化于1841~2768 Ma之间,并呈现出1960~2056 Ma(峰值2007 Ma)和2450~2540 Ma(峰值2494 Ma)两个相对集中的年龄峰值(图6b)。
来自青白口系下部独断山组的样品(ks_46)共测试分析了48个锆石(表2),其中,谐和度>95%的测点44个(图6c),锆石U_Pb年龄变化于1842~2786 Ma之间,并呈现了1956~2063 Ma(峰值2015 Ma)和2468~2542 Ma(峰值2501 Ma)两个相对集中的年龄峰值(图6d)。样品中,除了3个点的Th/U比值为0.29、0.36和0.01,其他所有年龄谐和锆石的Th/U比值均大于0.4,说明这些锆石大多是岩浆成因的。样品ks_46的锆石CL图像(图7a)显示,年轻一组(<2100 Ma)的锆石常呈次棱角状,多数锆石环带清晰。较老一组年龄(>2400 Ma)的锆石多呈圆状_次圆状(图7a),说明锆石经过了较长时间的风化剥蚀,这与其年龄特征相符。
切穿含矿地层的辉绿岩样品(KS_J02)中锆石U_Pb年龄分析点共计16个(表3)。部分锆石CL图像见图7b,除5个测点年龄明显不同外,其他11个测点相对集中(图8a)。最年轻一组锆石的206Pb/238U年龄加权平均值为(640.2±5.5) Ma(MSWD=0.79, n=11)(图8b)。样品中年龄协和的锆石Th/U比值除一粒为0.32外,其余均大于0.4。年轻一组锆石(<700 Ma)形态上呈次棱角状_棱角状,完整的锆石颗粒粒径较大,震荡环带清晰;部分锆石颗粒呈破碎状,粒径较小,但是从残留的锆石形态可以推断其未破碎之前应该具有较大的粒径,这些外部形态特征与同一样品中的年龄较老的一组锆石形成了鲜明对比。因而,结合锆石的外部形态特征、锆石内部清晰震荡环带特征及Th/U比值,笔者认为年轻一组的锆石应为原生的岩浆锆石,其谐和年龄代表了辉绿岩的侵位年龄。其他5个相对古老的锆石多数呈圆形_次圆状,磨圆度较高,应是经受长时间风化剥蚀搬运的结果,其年龄可能代表了岩浆上升过程中所捕获基底岩石的年龄(张英利等, 2011; 曹晓峰等, 2012)。
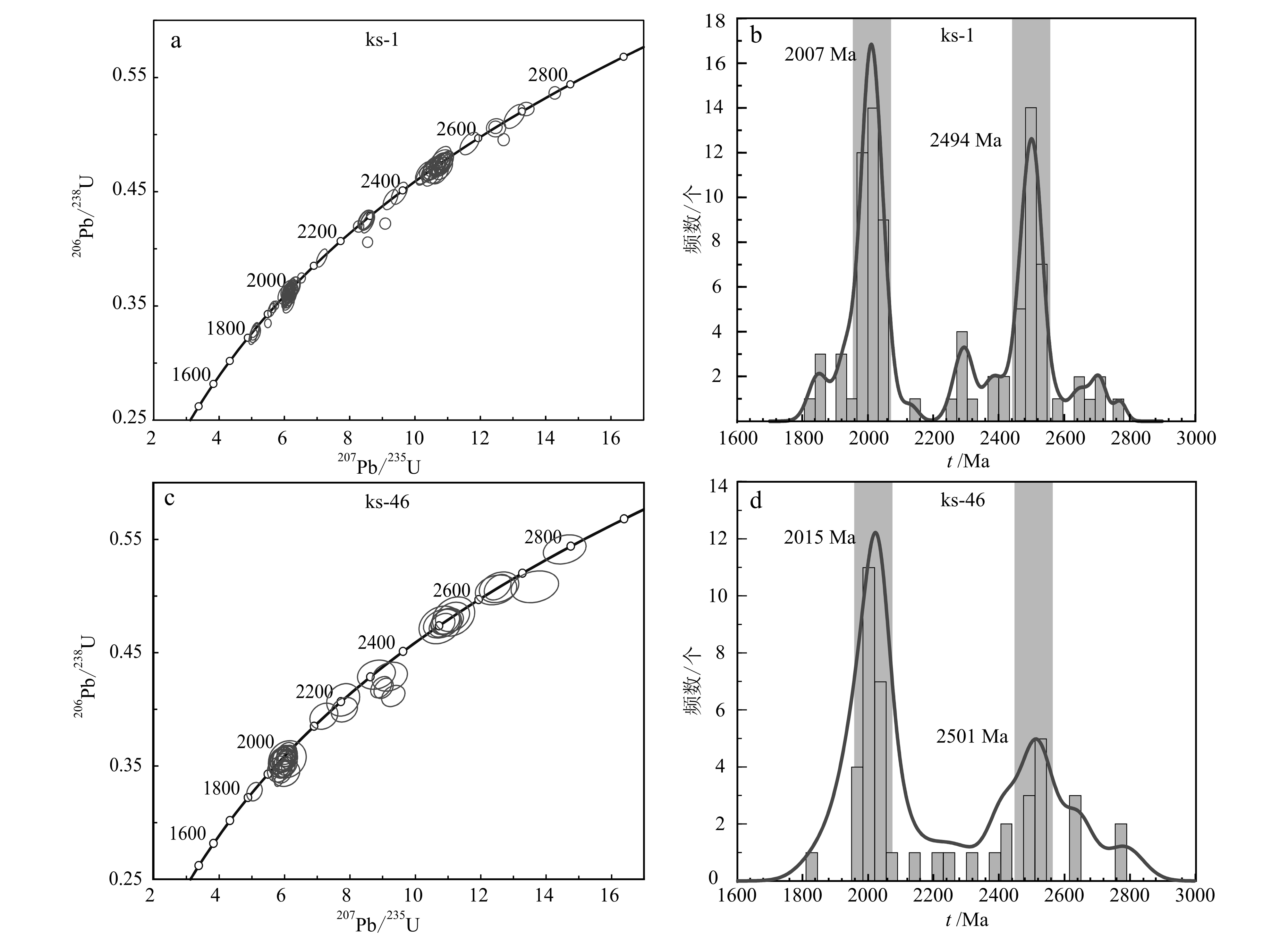
图 6 库鲁克赛铁矿铁矿石(a、b)和围岩(c、d)碎屑锆石U_Pb协和曲线图和年龄谱图
Fig. 6 U_Pb detrital zircon concordia diagram and and age spectra of ironstone (a, b) and surrounding rocks (c,d)
from Quruqsay iron formation
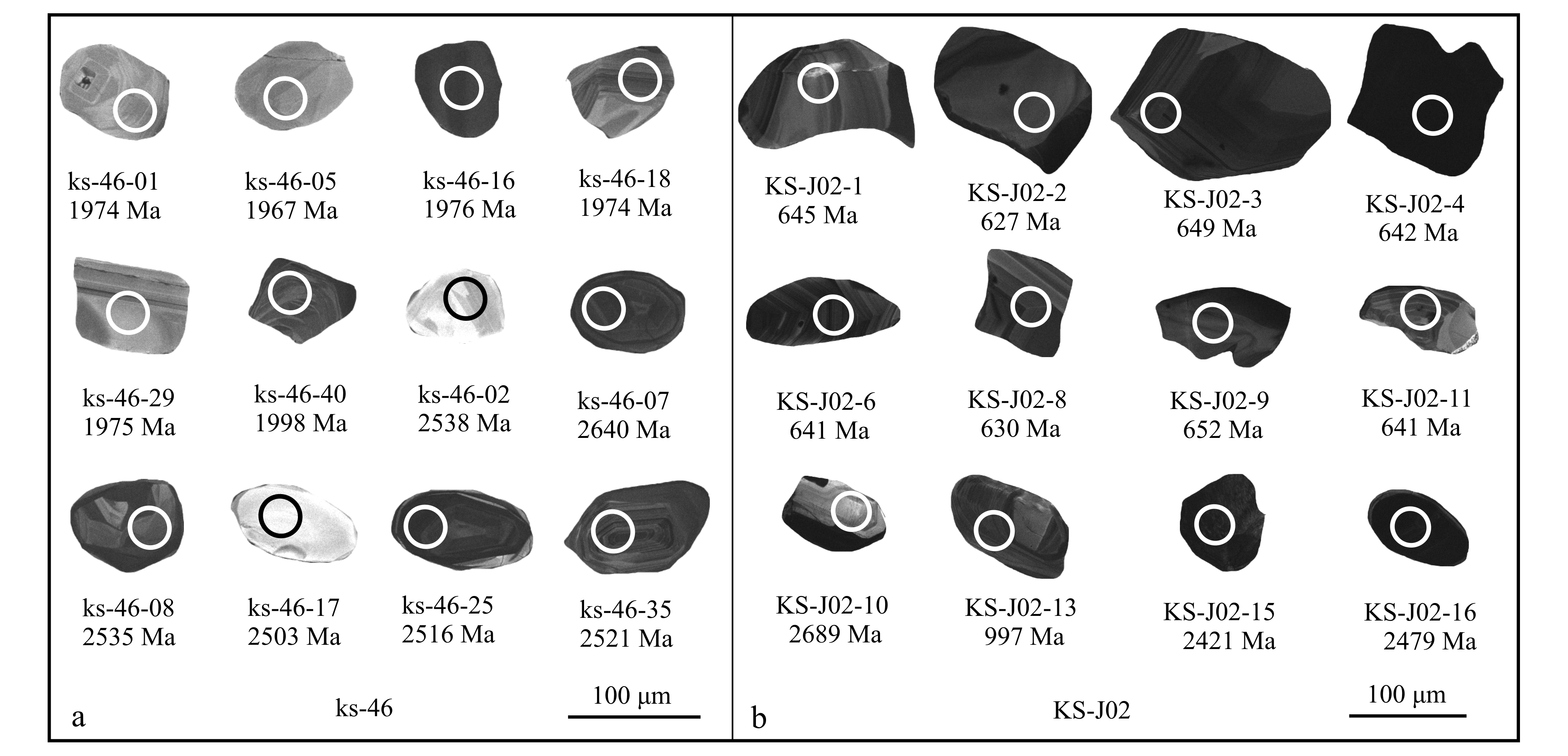
图 7 库鲁克赛铁矿矿石中部分碎屑锆石CL图像(a)和辉绿岩中部分碎屑锆石CL图像(b)
Fig. 7 Representative cathodoluminescence images of zircons from ironstone (a) and diabase dyke (b)
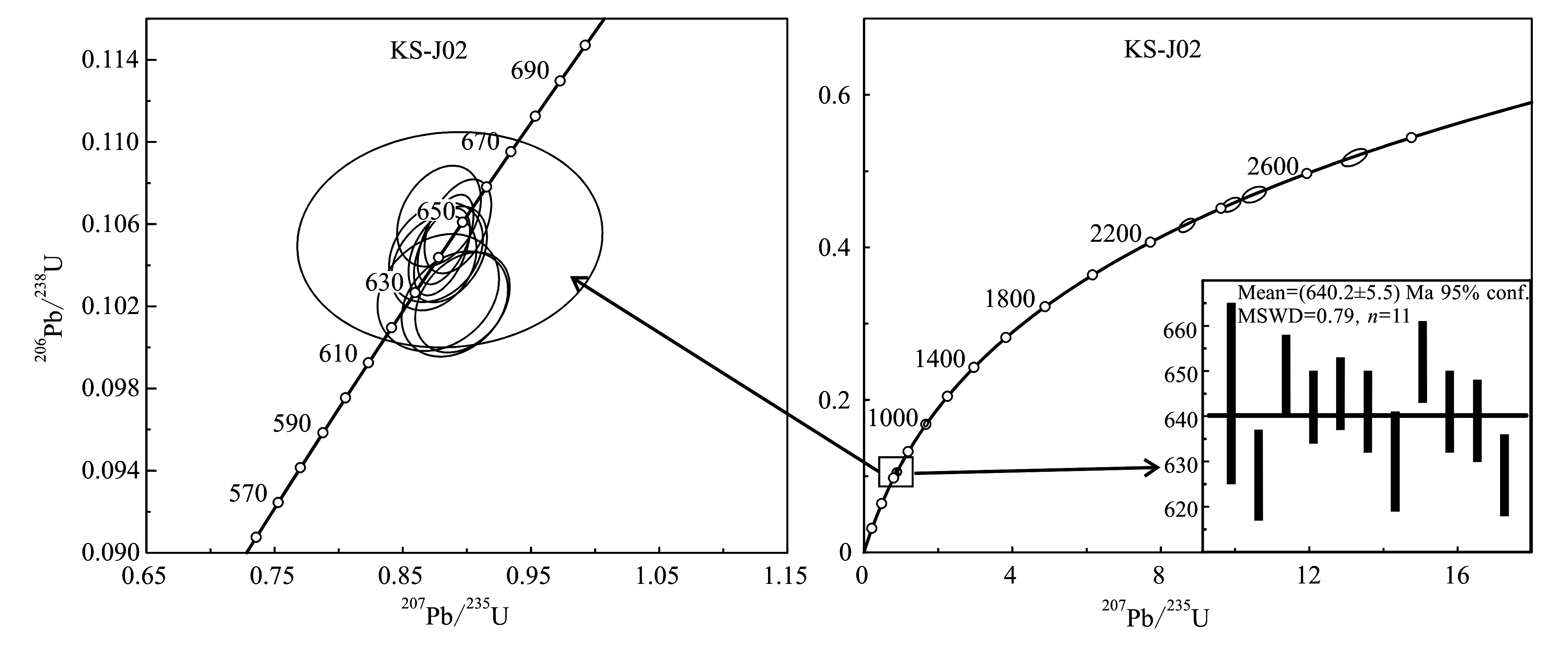
图 8 库鲁克赛铁矿区辉绿岩侵入体中锆石U_Pb协和曲线图
Fig. 8 U_Pb concordia diagrams for diabase dyke from Quruqsay iron formation
表 4 库鲁克赛铁矿铁矿石的主量元素测试结果
Table 4 Major element concentration of ironstones from Quruqsay iron formation
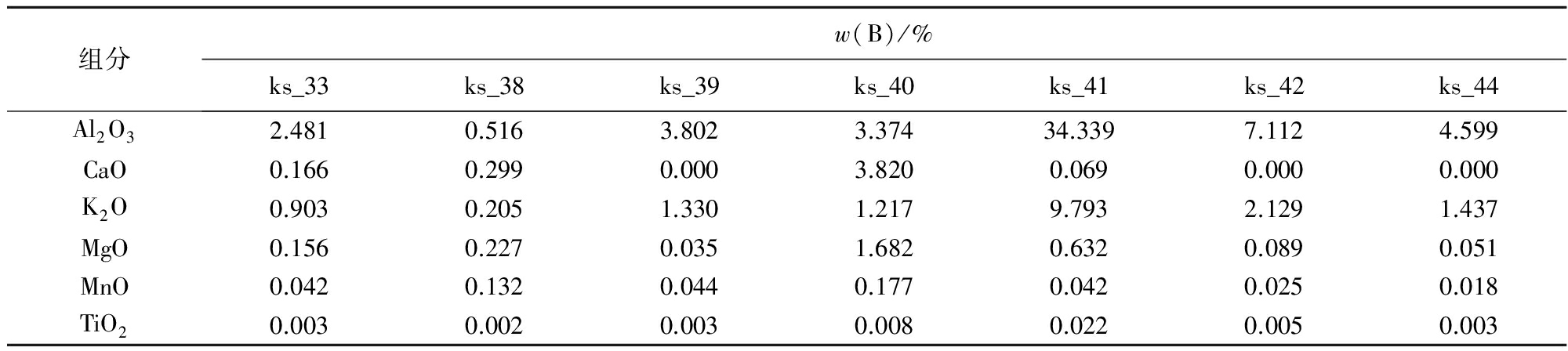
组分w(B)/%ks_33ks_38ks_39ks_40ks_41ks_42ks_44Al2O32.4810.5163.8023.37434.3397.1124.599CaO0.1660.2990.0003.8200.0690.0000.000K2O0.9030.2051.3301.2179.7932.1291.437MgO0.1560.2270.0351.6820.6320.0890.051MnO0.0420.1320.0440.1770.0420.0250.018TiO20.0030.0020.0030.0080.0220.0050.003
5.2 地球化学
本文主微量元素分析的样品共7个,均为采自矿体的铁矿石,岩性上因砾石含量不同而略有差别,具体编号和采样情况如图4所示,主量元素分析测试工作在南京大学表生地球化学教育部重点实验室进行。所用仪器为Thermo fisher生产的电感耦合等离子体发射光谱仪(ICP_OES),具体仪器型号为Icap6300。微量元素分析在南京聚谱检测科技有限公司进行,使用由安捷伦科技公司制造的型号为Agilent7700x的电感耦合等离子体质谱仪测量,内精度为3%。7个样品的主量元素测试结果列于表4,微量元素测试分析结果列于表5。
ICP_OES的测试结果显示,样品中w(Al2O3)介于0.5%~35%,除去偏差较大的样品(ks_41),得到平均值为3.65%。岩石的w(K2O+MgO+CaO)介于0.73%~10.49%,除去明显高于其他样品编号为ks_41的样品,得到平均值为2.29%。
微量元素中测试样品中,w(Sc)范围为(2.29~33.60)×10-6,均值为9.60×10-6,除去十分异常的ks_41,w(Sc)平均值为5.61×10-6。w(Zr)普遍较高,除样品ks_38(w(Zr)为7.81×10-6)外,其余样品均高于60×10-6(变化范围为(62.11~355.11)×10-6,均值为165.26×10-6)。Zn/Co比值介于0.54~1.42,均值0.85。
本区样品的稀土元素总量偏低,且变化范围较大(34.75~340.95)×10-6,平均值为138.58×10-6。轻、重稀土元素比值通常用(La/YbSN)来表征,变化范围为0.16~1.12,均值为0.69。样品的变化范围为0.88~1.14,均值为1.05,在(REE+Y)的配分模式图(图9b)中呈现出铕异常不明显的特征。
变化范围较大(0.74~1.24),在Bau等(1996)的Ce异常判断图解9b)(图中,2个样品表现出明显的Ce负异常,1个样品呈现出Ce的正异常,其他样品Ce异常不显著。样品的Y/Ho比值介于28.48~38.91,均值为33.16,其中,除ks_41的Y/Ho比值与球粒陨石(28;Bau et al., 1997; 1999)相近外,其余样品的值均高于球粒陨石和澳大利亚页岩的Y/Ho比值(26;Pourmand et al., 2011)的值。
表 5 库鲁克赛铁矿铁矿石的微量元素测试结果
Table 5 Trace element concentration of ironstones from Quruqsay iron formation
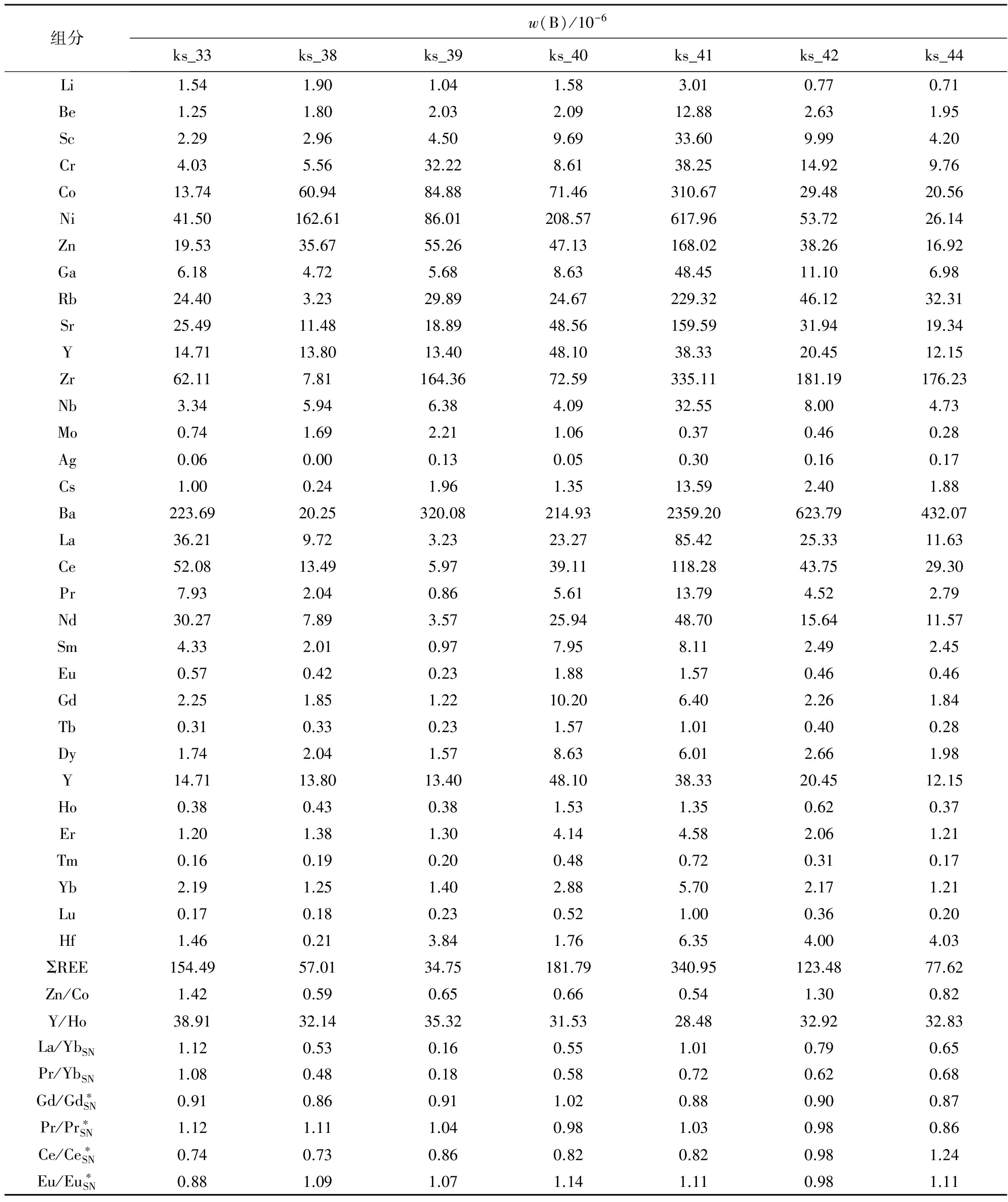
组分w(B)/10-6ks_33ks_38ks_39ks_40ks_41ks_42ks_44Li1.541.901.041.583.010.770.71Be1.251.802.032.0912.882.631.95Sc2.292.964.509.6933.609.994.20Cr4.035.5632.228.6138.2514.929.76Co13.7460.9484.8871.46310.6729.4820.56Ni41.50162.6186.01208.57617.9653.7226.14Zn19.5335.6755.2647.13168.0238.2616.92Ga6.184.725.688.6348.4511.106.98Rb24.403.2329.8924.67229.3246.1232.31Sr25.4911.4818.8948.56159.5931.9419.34Y14.7113.8013.4048.1038.3320.4512.15Zr62.117.81164.3672.59335.11181.19176.23Nb3.345.946.384.0932.558.004.73Mo0.741.692.211.060.370.460.28Ag0.060.000.130.050.300.160.17Cs1.000.241.961.3513.592.401.88Ba223.6920.25320.08214.932359.20623.79432.07La36.219.723.2323.2785.4225.3311.63Ce52.0813.495.9739.11118.2843.7529.30Pr7.932.040.865.6113.794.522.79Nd30.277.893.5725.9448.7015.6411.57Sm4.332.010.977.958.112.492.45Eu0.570.420.231.881.570.460.46Gd2.251.851.2210.206.402.261.84Tb0.310.330.231.571.010.400.28Dy1.742.041.578.636.012.661.98Y14.7113.8013.4048.1038.3320.4512.15Ho0.380.430.381.531.350.620.37Er1.201.381.304.144.582.061.21Tm0.160.190.200.480.720.310.17Yb2.191.251.402.885.702.171.21Lu0.170.180.230.521.000.360.20Hf1.460.213.841.766.354.004.03ΣREE154.4957.0134.75181.79340.95123.4877.62Zn/Co1.420.590.650.660.541.300.82Y/Ho38.9132.1435.3231.5328.4832.9232.83La/YbSN1.120.530.160.551.010.790.65Pr/YbSN1.080.480.180.580.720.620.68Gd/Gd∗SN0.910.860.911.020.880.900.87Pr/Pr∗SN1.121.111.040.981.030.980.86Ce/Ce∗SN0.740.730.860.820.820.981.24Eu/Eu∗SN0.881.091.071.141.110.981.11
注: 比值单位为1。稀土元素页岩标准化简写为“SN"(页岩为前寒武纪澳大利亚页岩,简写为“PAAS"),数据来自Pourmand et al., 2011。 其中,
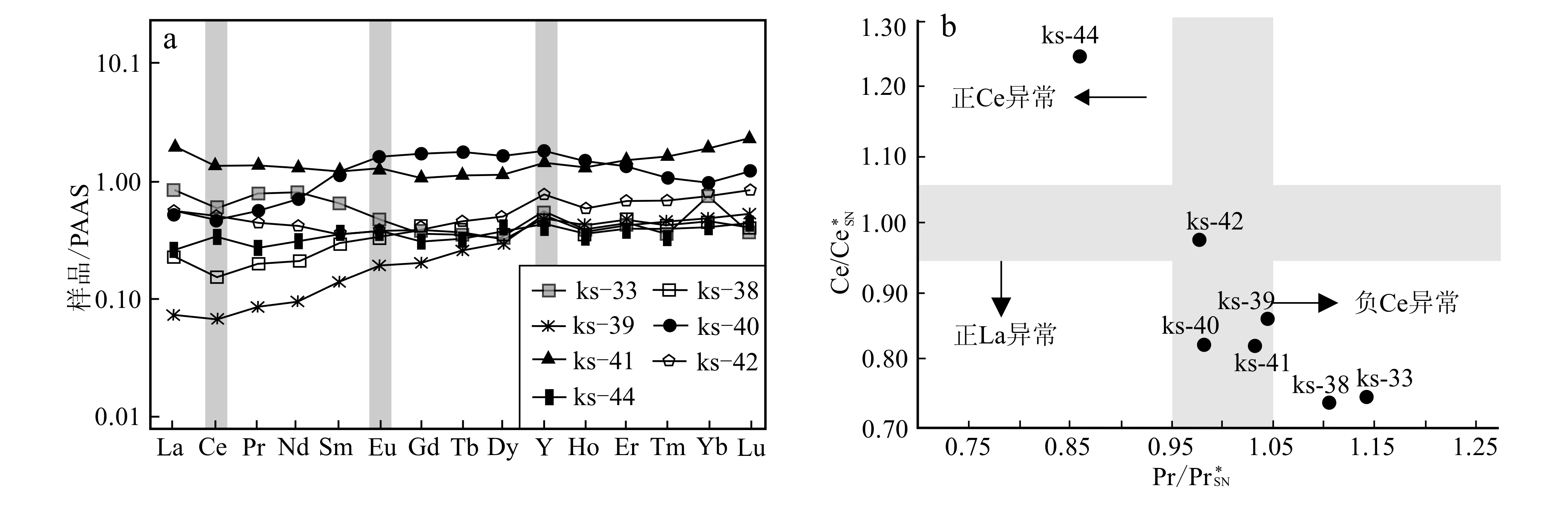
图 9 库鲁克赛铁矿(REE+Y)配分曲线(a)及Ce/Ce*-Pr/Pr*判别图解(b)(标准化数据采用Pourmand et al., 2011)
Fig. 9 PAAS_normalized (REE+Y) patterns for ironstone from the Quruqsay iron formation (a) and Ce/Ce*_Pr/Pr* plot (b) (normalization values after Pourmand et al., 2011)
6 讨 论
6.1 沉积时代的限定
采自铁矿体的富铁砾岩样品(ks_1)及青白口系独断山组砂岩(ks_46)的碎屑锆石U_Pb年龄变化范围分别为1841~2768 Ma和1842~2786 Ma。富铁砾岩的碎屑锆石年龄谱与其下伏的青白口系年龄谱基本一致,其最小的年龄值分别为1841 Ma和1842 Ma,只能代表最大沉积年龄。本次获得侵位于铁矿体中辉绿岩(KS_J02)的锆石U_Pb年龄((640±5.5) Ma)可以约束地层的最小年龄。
前人根据库鲁克赛铁矿下部的地层为青白口系横断山组,将其形成时代划归为青白纪(新疆地质矿产局,1995)。由于构造作用的影响,矿区内新元古界总体褶皱弯曲形成了一个小型的向斜构造。库鲁克赛铁矿的赋存地层位于这一向斜的两翼(图3),多数矿体呈透镜状被青白系横断山组白云岩地层包围,少数矿体呈似层状角度不整合于青白系横断山组白云岩地层(高振家等, 1984; 1985)之上。向斜的核部为南华系—震旦系库鲁克塔格群火山_沉积岩系。
本区新元古代地层中的碎屑锆石最小年龄峰值为2.0 Ga左右,缺乏中元古代及新元古代早期锆石年龄的记录。这一结果与前人对库鲁克塔格地区新元古代晚期地层中的碎屑锆石年代学研究结果一致,即缺乏8.0~18亿年之间的锆石年龄记录(何景文等, 2015; 高林志等,2013; 张英利等, 2010),这可能是因为库鲁克塔格地块在这一时期的岩浆热事件较弱,没有大量新生地壳形成。此外,研究区范围内震旦系分布广泛,冰碛岩序列发育(Norin, 1935; 高振家等, 1984; 1985; 2003; 高林志等, 2010; 2013)。库鲁克赛铁矿本身具有冰碛岩特征(图5e),其下部不整合覆盖于青白口系白云岩之上,与贝义西组岩性组合特征相同。此外,高振家等(1984)和高林志等(2010)将该套地层划归为贝义西组冰碛岩地层,因此,在综合考虑地层特征和前人研究成果的基础上,笔者将库鲁克赛铁矿的形成时期划为库鲁克塔格地区贝义西冰期,其形成时代应介于(768±10) Ma~(740±7) Ma之间(Xu et al., 2005; 2009; 徐备等, 2008; 高林志等, 2013)。
6.2 物质来源
库鲁克赛铁矿的含矿建造为一套铁质胶结的砾岩。岩性因砾石含量不同而略有差别,含铁砾岩主要为碎屑结构,碎屑物质粒径变化极大。镜下观察显示,矿石中的碎屑主要为石英砂岩岩屑、燧石和单颗粒石英等,胶结物几乎均为铁氧化物。矿石样品的主量元素中,w(K2O+MgO+CaO)值普遍小于5%,与镜下观察未发现方解石、长石和黑云母等相关矿物,而仅以石英和铁氧化物相存在的现象一致。样品中的碎屑物质混杂堆积,分选度和磨圆度均很差,且成分单一,反应了其近源堆积的特征。赋矿地层下伏的青白口系独断山组主要岩性为变质石英砂岩(图10a)、变质长石石英砂岩(图10b)、细粒变质石英砂岩(10c)和变质长石杂砂岩(图10d)。该组岩性与矿石中的碎屑(图10e)组分具有较强的一致性。
此外,ks_1(采自矿体)和ks_46(采自独断山组)碎屑锆石年龄谱值也非常一致,且具有2个极为接近的年龄峰值(图6b、d),暗示了两者的锆石来源也具有一致性。因此,本文推测,库鲁克赛铁矿中的碎屑物质来自其下伏的基底地层;可能与青白口系帕尔岗塔格群独断山组有关。
铁建造中铁的来源主要有3种:海底的热液流体、大陆风化搬运_生物再活化和两者的混合作用(Li et al., 2015)。Eu异常在大洋洋中脊热液体系中较为常见,中高温的海底热液表现出明显的Eu正异常(Michard et al., 1986; Stern et al., 2013; 王祥东等, 2014)。库鲁克赛铁矿整体上缺乏明显的Eu正异常(Eu/Eu*<1.15),这与Basta等(2011)研究的Wadi Kareim和Um Anab条带状铁建造中铕异常值(1.18±0.05)具有一致性,反应出在铁矿的形成过程中,缺乏中_高温热液流体的加入。
微量元素中,Sc、Zr含量和Zn/Co比值通常被用来作为指示铁质来源的重要判别指标。高的Sc、Zr含量、低Zn/Co比值被认为是陆壳来源的重要证据(Halverson et al., 2011; Sial et al., 2015; Stern et al., 2013)。来自库鲁克赛铁矿体的样品具有较高的w(Sc)(平均值8.5×10-6)、w(Zr)(均值133.49×10-6,除ks_38之外的均值为151.45×10-6)和低的Zn/Co比值(均值为0.85, n=7)(图11a~c),暗示库鲁克赛铁矿的铁物质主要来自于风化陆壳。
稀土元素的地球化学特征已经成为研究沉积型铁矿床成矿过程和铁物质来源的重要手段(Halverson et al., 2011; Stern et al., 2013)。
Y/Ho比值是区别海水和其他沉积环境的重要指标。前人的研究发现,陆壳岩石和洋中脊高温热液的Y/Ho比值约为28,在风化过程或者海水搬运过程中Y/Ho比值会出现明显的升高,现代海水的Y/Ho比值高达43~80,古元古代表面海水的Y/Ho比值约为65 (Bau et al., 1997; 1999)。库鲁克赛铁矿Y/Ho比值介于27~40,平均为32.4(图11e),接近陆壳岩石和洋中脊高温热液的Y/Ho比值,明显低于海水的Y/Ho比值,反映出海水本身的铁物质加入是十分匮乏的。考虑到样品中碎屑物质的大量存在以及主量元素和Eu异常的测试结果,Y/Ho比值应该更多的继承于陆壳岩石的风化。Ce负异常是现代富氧海水的主要特征之一 (Zhang et al., 1996;Alibo et al., 1999)。但王一先等(1996)研究南极菲尔德斯半岛湖泊沉积物时发现,湖泊下部的冰碛岩地层中Ce异常不明显(0.87~1.15)。陈志华等(2015)在研究南极半岛东北部海域表层沉积物稀土元素特征时亦发现冰川前缘冰水沉积物中Ce异常不明显的特征。而库鲁克赛铁矿自下而上,整体上轻稀土元素相对重稀土元素亏损、Ce异常不明显(图11d),可能反映的并不是当时海洋表层水体的特征,而是冰下通道内缺氧冰下水体与富氧海水混合水体的特征。
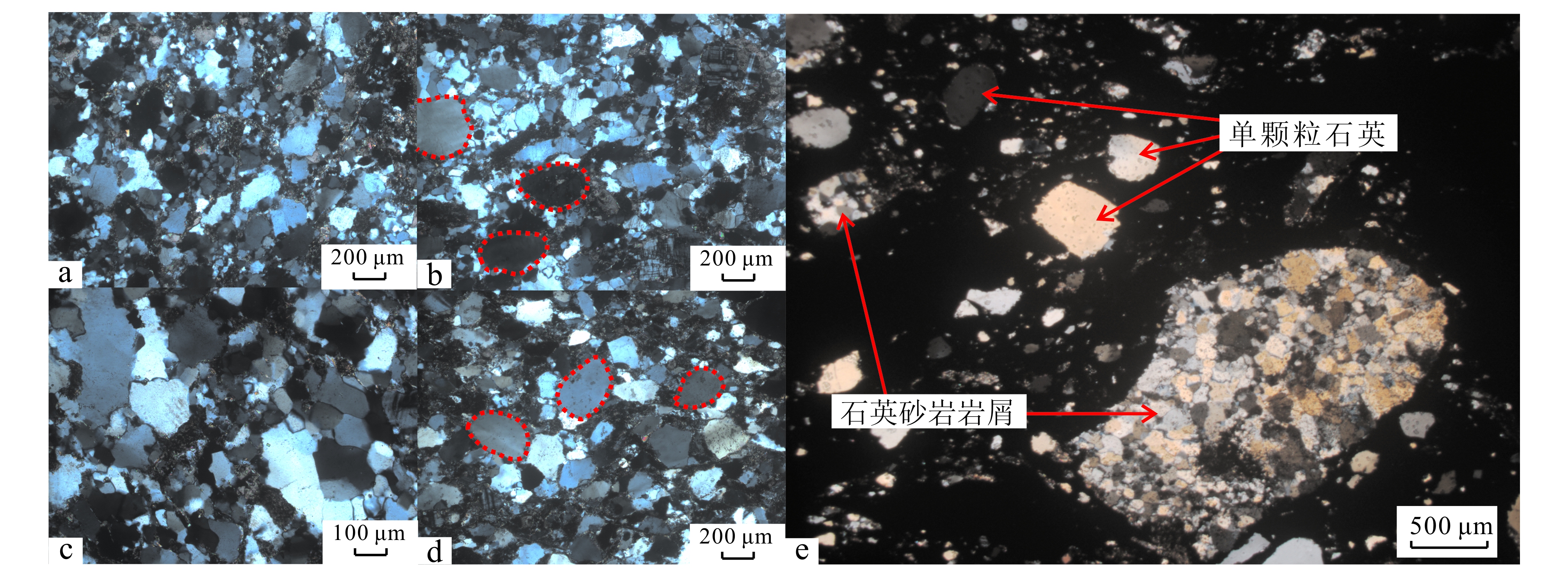
图 10 青白口系独断山组代表性岩石(a~d)与库鲁克赛铁矿中碎屑组分(e)镜下岩相对比图(正交偏光)
a. 镶嵌式胶结,石英具波状消光的变质石英砂岩; b. 单颗粒石英磨圆度较好的变质长石石英砂岩; c. 具镶嵌式胶结和波状消光的细粒变质石英砂岩; d. 点接触关系,单颗粒石英磨圆度较好的变质长石石英杂砂岩; e. 碎屑成分主要由石英和石英砂岩碎屑组成的铁矿石(样品ks_38): 单颗粒石英一般粒径较大且具有较好的磨圆度;石英砂岩岩屑中矿物颗粒主要为石英,通常呈镶嵌式胶结,具波状消光
Fig. 10 Lithofacies contrast diagram between representative samples of Qingbaikouan Group (a~d) and the detrital debris in ironstone (e) (crossed nicols)
a. Mosaic cementation and wavy extinction in deformed quartz sandstone; b. Rounded quartz grains in deformed feldspar quartz sandstone; c. Mosaic cementation and wavy extinction in fine_grained quartz sandstone; d. Point contact features and some rounded quartz in feldspar quartz greywacke; e. Ferruginous conglomerate: Gravel is mainly composed of single quartz grains and quartz sandstone debris, single quartz grains are well_rounded and the sandstone debris has mosaic cementation and wavy extinction
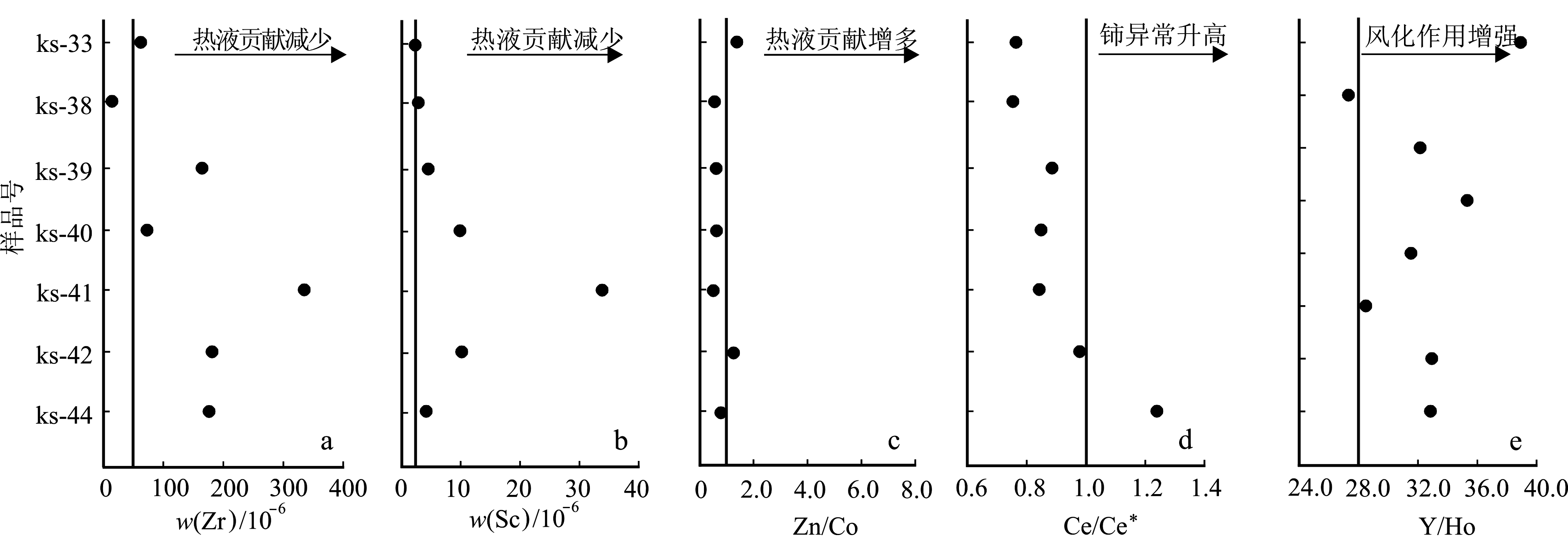
图 11 库鲁克赛铁矿各样品元素含量与元素比值变化情况
Fig. 11 Variation of elemental abundances and ratios in the ore samples
综合以上分析,本文认为库鲁克赛铁矿是冰川下部陆壳风化产物搬运入海,进而沉积成矿的结果,成矿物质应主要是陆壳风化的产物。
6.3 沉积环境与Fe2+沉淀机制
新元古代铁建造的成因模式一直是学术界讨论的焦点,诸多针对新元古界铁矿建造的成因模式逐渐被提出,如裂谷沉积喷流模式(Sedimentary exhalative_rifting; Eyles et al., 2004)、雪球地球模式(Snowball Earth; Klein et al., 1993; Hoffman et al., 2002; Halverson et al., 2011)、亚冰期沉积模式(Anoxic sub_glacial outwash; Mikucki et al., 2009; Lechte et al., 2016)、缺硫模式(Sulfur limitation; Kump et al., 2005)、大陆剥蚀模式(Continental resurfacing; Swanson_Hysell et al., 2010)和局限洋盆模式(Silled basins; Baldwin et al., 2012)等。上述成因模式之间多具有一定的相关性,并可被概略总结为冰期模式(syn_glacial model)和裂解模式(syn_rifting model)。
新元古代铁建造是在地球氧气含量相对较高的环境下形成的(Canfield, 2005; Frei et al., 2009), 大多数新元古代条带状铁建造与冰期相关,多以层状铁质粉砂岩的形式存在,而不同于以磁铁矿+碧玉条带为特征的太古代—古元古代的铁建造(Cox et al., 2013)。在矿物组成方面,不同于太古代—古元古代条带状铁建造,大多数新元古代铁建造的矿石矿物以赤铁矿的形式存在(Klein et al., 1993; 2004; Halverson et al., 2011; Cox et al., 2013; Angerer et al., 2016);仅少数铁建造矿石矿物以磁铁矿为主(余志庆等, 1989; Volkert et al., 2010; Freitas et al., 2011; 李厚民, 2012; 李志红等, 2014; Lei et al., 2016)。作为太古代—古元古代铁矿中普遍产出的含铁矿物,磁铁矿通常被认为是在成岩过程中形成的,与Fe(OH)3和有机质的氧化还原反应有关(Johnson et al., 2008),反映了沉积过程中海洋较高的初级生产力(Li et al., 2015)。库鲁克赛铁矿的矿石矿物为赤铁矿,这指示出Fe2+氧化沉淀的位置处,海水的初级生产力不高,没有大规模有机质与Fe(OH)3共沉淀。因此,氧化Fe2+的氧化剂有较大可能是溶解于海水中的自由氧,而非生物作用。
Black等(1965)研究泰勒冰川下部水体中的生物群体时,发现该冰川流出口处有大量红色液体流出(血瀑布,Blood falls)。随着研究的深入(Angino et al., 1964; Keys, 1979; Lyons et al., 1998),前人发现液体呈红色是由于含有较高铁氧化物的结果。Keys(1979)随后指出,冰下水体刚流出冰川流出口时并不可见,仅当与外界空气接触时,水体中的二价铁被氧化才显示出红色特征。之后,被氧化的三价铁的氢氧化物沉积下来,以胶结物的形式存在于基底碎屑之间。Mikucki等(2009)认为该富铁、高盐、贫氧、含有较多微生物的水体被氧化沉淀的过程可与新元古代铁建造的形成过程类比。Hoffman等(2011)也认为冰川覆盖下的海水可能像血瀑布附近的冰下水体一样,具有贫氧、高盐、富铁的特征。尽管Cox等(2013)认为规模较大的Rapitan型铁建造不可能以“血瀑布”形成方式产生,但是对于规模较小、分布面积较为局限的铁建造,可参考“血瀑布”形成过程。
6.4 成矿模型
库鲁克赛铁矿形成于新元古代青白口纪末期—南华纪早期(介于(768±10) Ma~(740±7) Ma之间),赋矿地层为含铁砾岩,矿体分布面积较为局限,均以透镜状、似层状形式产出。矿石矿物主要是以胶结物形式存在的赤铁矿,脉石矿物为粒径大小不一的石英、石英砂岩碎屑,成分单一,无分选和磨圆,反应出冰川前缘冰水沉积物的特征。锆石年龄谱值对比和岩相学研究表明,铁矿床中的碎屑物质主要来自于青白口系下部独断山组石英砂岩地层。此外,库鲁克赛铁矿较高的Sc、Zr含量、低Zn/Co比值、Eu异常不明显的特征以及与陆壳岩石较为相近的Y/Ho比值,反映出铁物质的陆源特征。矿层自上向下Ce异常不明显,结合矿石矿物几乎全部为赤铁矿的事实,这可能反映出成矿过程中亚铁离子快速氧化沉淀而没有出现明显分异的特征。
综合上述分析,本文提出了库鲁克赛铁矿床的冰川前缘沉积模型(图12)。新元古代成冰纪,库鲁克塔格地块总体为冰川覆盖,并发育了多期冰碛岩(Norin, 1935; 高振家等, 2003)。由于巨大的冰盖压蚀作用,冰川下部形成了一定规模的冰下水体。因长时间与大气隔绝,冰下水体呈现出贫氧的特征(Mikucki et al., 2004a; 2004b; Le Heron et al., 2009)。在贫氧环境下,冰下水体中亚铁离子溶解度和含量逐渐升高(Mikucki et al., 2009)。由于外部气候的变化,至间冰期时,冰川开始融化,富含亚铁离子的贫氧冰下水体沿冰川峡谷和冰下河流等冰下通道向无冰川覆盖区溢出。当溢出水体与外界相对富氧的海水和大气进行接触时,其中的亚铁离子被迅速氧化形成三价铁的化合物,三价铁的化合物在水中的溶解度较低,进而以胶体的形式在冰川流出口较近位置沉积下来。冰下水体沿冰下通道溢出时,其所携带的基底(青白口纪帕尔岗塔格群的石英砂岩和白云岩)碎屑物质也相应的沉积下来。由于搬运距离较短,基底碎屑物质未经分选和磨圆,从而形成了库鲁克赛铁质胶结的冰碛砾岩型铁矿。这一过程类似于南极泰勒冰川前缘“血瀑布"的形成过程。
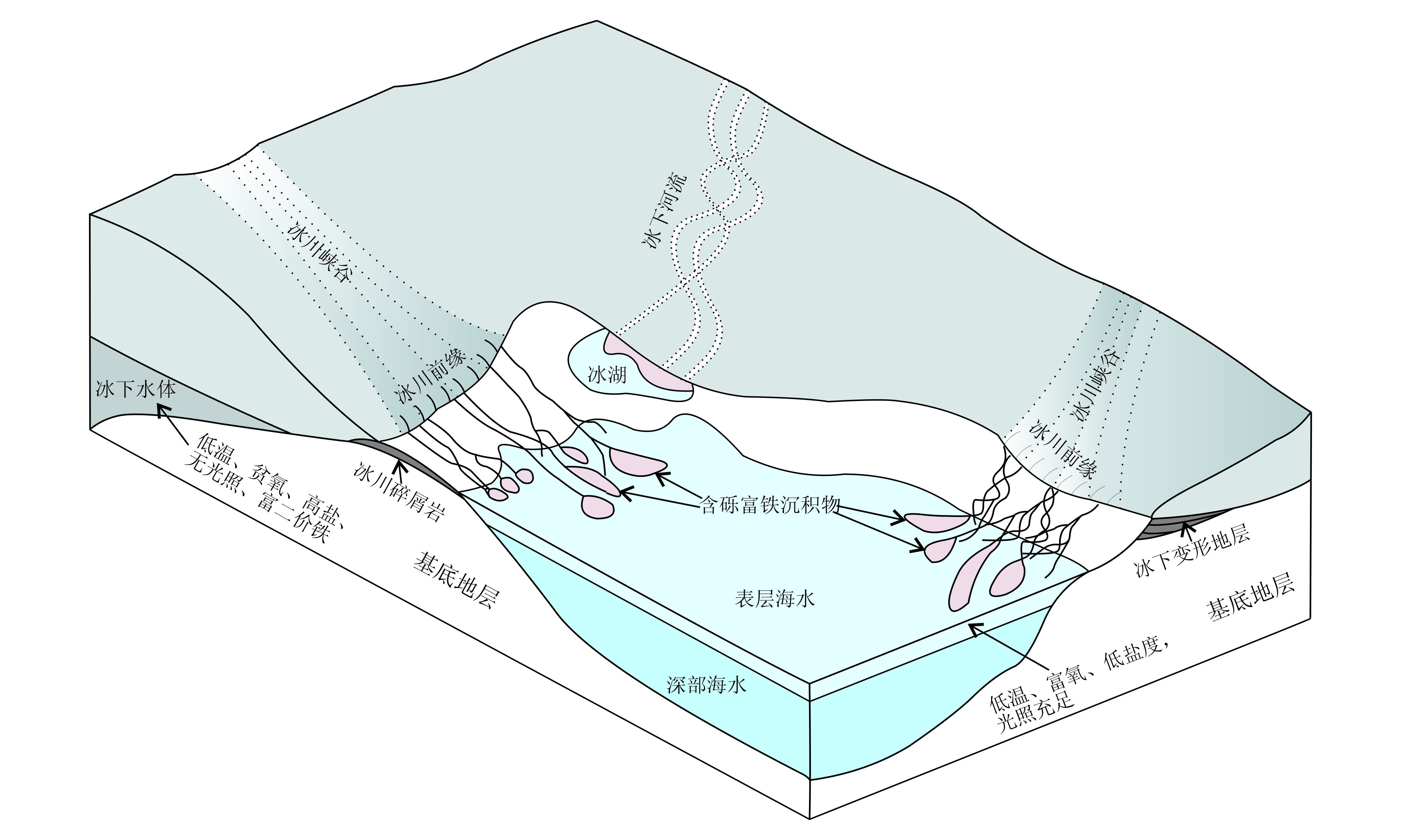
图 12 库鲁克塔格地区部分新元古代赤铁矿床形成机制模型
Fig. 12 Formation mechanism model for some Neoproterozoic iron deposits in the Quruqtagh block
7 结 论
(1) 库鲁克赛铁矿赋存于青白口系横断山组白云岩之上、南华系—震旦系库鲁克塔格群之中;铁矿石中的碎屑锆石U_Pb年龄结果揭示出赋矿地层的形成时代晚于18亿年;侵入于赋矿地层的辉绿岩脉的岩浆锆石年龄显示岩脉侵位时代为(640.2±5.5) Ma。因此,库鲁克赛铁矿应形成于新元古代青白口纪末期—南华纪早期,与全球新元古代早期成铁事件一致。
(2) 矿石中的砾石大小混杂、磨圆度分选均差,可见有大块石英砂岩岩屑和燧石团块;部分矿石中还见有坠石,具有冰水沉积物的特征。结合库鲁克塔格地块广泛发育的与全球4次冰期相对应的冰碛岩,认为库鲁克赛铁矿含矿地层与成冰纪的冰川事件有关,为冰碛砾岩型铁矿床。
(3) 库鲁克赛铁矿中的碎屑物质呈混杂堆积,分选度和磨圆度均差反应出其近源堆积的特征;样品ks_1(采自矿体)和ks_46(采自独断山组)碎屑锆石年龄谱非常一致,揭示出铁矿石中碎屑物质与下伏青白口系独断山组地层的亲缘性。矿石样品具高的Sc、Zr和低的Zn/Co比值特征,缺乏明显的Eu正异常、Y/Ho比值介于27~40值,进一步表明矿床的成矿物质应主要来自于大陆地壳的风化。Ce异常不明显的特征可能与亚铁离子快速氧化沉淀而没有出现明显分异有关。
(4) 库鲁克赛铁矿的形成与成冰纪间冰期冰水沉积作用有关。来自冰下水体,从冰下通道中流出的富铁、贫氧、高盐的水溶液与外界富氧的海水混合时;亚铁离子发生快速氧化沉淀并胶结冰水中的近源砾石,进而形成了此种富铁砾岩型铁矿体。
志 谢 野外工作中得到新疆地矿局同仁的大力支持和帮助,新疆地调院董连慧、屈迅、赵同阳和石福品,东华理工大学黎广荣博士和夏芝广,南京大学王小敏和赵书高参与了野外工作,在此谨志谢忱!
References
Alibo D S and Nozaki Y. 1999. Rare earth elements in seawater: Particle association, shale northwestern, and Ce oxidation[J]. Geochimica et Cosmochimica Acta, 63 (3_4): 363_372.
Andersen T. 2002. Correction of common lead in U_Pb analyses that do not report 204Pb[J]. Chemical Geology, 192(1_2): 59_79.
Angerer T, Hagemann S G, Walde D H G, Halverson G P and Boyce A J. 2016. Multiple metal sources in the glaciomarine facies of the Neoproterozoic Jacadigo iron formation in the “Santa Cruz deposit", Corumbá, Brazil[J]. Precambrian Research, 275: 369_393.
Angino E E, Armitage K B and Tash J C. 1964. Physicochemical limnology of Lake Bonney, Antarctica[J]. Limnology and Oceanography, 9(2): 207_217.
Baldwin G J, Turner E C and Kamber B S. 2012. A new depositional model for glaciogenic Neoproterozoic iron formation: Insights from the chemostratigraphy and basin configuration of the Rapitan iron formation[J]. Earth Science, 49(2): 455_476.
Basta F F, Maurice A E, Fontbote L and Favarger P Y. 2011. Petrology and geochemistry of the banded iron formation (BIF) of Wadi Karim and Um Anab, eastern desert, Egypt: Implications for the origin of Neoproterozoic BIF[J]. Precambrian Research, 187(3): 277_292.
Bau M and Dulski P. 1996. Distribution of yttrium and rare-earth elements in the Penge and Kuruman iron-formations, Transvaal Super Group, South Africa[J]. Precambrian Research, 79(1-2): 37-55.
Bau M, Moller P and Dulski P. 1997. Yttrium and lanthanides in eastern Mediterranean seawater and their fractionation during redox_cycling[J]. Marine Chemistry, 56(1_2): 123_131.
Bau M and Dulski P. 1999. Comparing yttrium and rare earths in hydrothermal fluids from the Mid_Atlantic Ridge: Implications for Y and REE behavior during near_vent mixing and for the Y/Ho ratio of Proterozoic seawater[J]. Chemical Geology, 155(1_2): 77_90.
Bekker A, Slack J F, Planavsky N, Krape B, Hofmann A, Konhauser K O, Rouxel O and Planavsky J. 2010. Iron formation: The sedimentary product of a complex interplay among mantle, tectonic, oceanic, and biospheric processes[J]. Econ. Geol., 105(3): 467_508.
Black R F, Jackson M L and Berg T E. 1965. Saline discharge from Taylor Glacier, Victoria Land, Antarctica[J]. Journal of Geology. 73(1): 175_181.
Canfield D E and Teske A. 1996. Late Proterozoic rise in atmospheric oxygen concentration inferred from phylogenetic and sulphur_isotope studies[J]. Nature, 382(6587): 127_132.
Canfield D E. 2005. The Early history of atmospheric oxygen: Homage to Robert M. Garrels[J]. Annual Review of Earth and Planetary Sciences, 33(1): 1_36.
Canfield D E, Poulton S W and Narbonne G M. 2007. Late Neoproterozoic deep_ocean oxygenation and the rise of animal life[J]. Science, 315(5808): 92_95.
Cao X F, Lü X B, Gao X, Liu Y G, Zhu J, Chen C, Wang Y J, Liu Z and Zhang B. 2012. Magmatic activities and tectonic evolution of Xinjiang Precambrian Quruqtagh Block, NW China[J]. Xinjiang Geology, 30(4): 384_391(in Chinese with English abstract).
Carlos A S, Sonia M B, Alcides N S and Francisco J R. 2007. Geochemistry and genesis of the banded iron formations of the Cauê Formation, Quadrilátero Ferrífero, Minas Gerais, Brazil[J]. Precambrian Research, 152(3_4): 170_206.
Chen Z H, Huang Y H, Tang Z, Wang H Z, Ge S L, Fang X S, Han X B, Wang A J, Wu L and Zhu Z M. 2015. Rare earth elements in the offshore surface sediments of the northeastern Antarctic peninsula and their implications for provenance[J]. Marine Geology and Quaternary Geology, 35(3): 145_155(in Chinese with English abstract).
Cloud P. 1973. Paleoecological significance of the banded iron formation[J]. Econ. Geol., 68(7): 1135_1143.
Cox G M, Halverson G P, Minarik W G, Le Heron D P, Macdonald F A, Bellefroid E J and Strauss J V. 2013. Neoproterozoic iron formation: An evaluation of its temporal, environmental and tectonic significance[J]. Chemical Geology, 362(1): 232_249.
Derry L A and Jacobsen S B. 1990. The chemical evolution of Precambrian seawater: Evidence from REEs in banded iron formations[J]. Geochimica et Cosmochimica Acta, 54(11): 2965_2977.
Eyles N and Januszczak N. 2004. ‘Zipper_rift’: A tectonic model for Neoproterozoic glaciations during the breakup of Rodinia after 750 Ma[J]. Earth_Science Reviews, 65(1): 1_73.
Feng B Z, Zhou Y W and Chi S F. 1995. Presinian Geology, Precious and nonferrous metal deposits in Quruqtagh area, Xinjiang, China[M]. Beijing: Geological Publishing House (in Chinese).
Fike D A, Grotzinger J P, Pratt L L and Summons R E. 2007. Ediacaran oxidation and biotic evolution_reply[J]. Nature, 450(7170):17_18.
Frei R, Gaucher C, Poulton S W and Canfield D E. 2009. Fluctuations in precambrian atmospheric oxygenation recorded by chromium isotopes[J]. Nature, 461(7261): 250_253.
Freitas B T, Warren L, Boggiani P C, De Almeida R P and Piacentini T. 2011. Tectono_sedimentary evolution of the Neoproterozoic BIF_bearing Jacadigo Group, SW_Brazil[J]. Sedimentary Geology, 238 (1_2): 48_70.
Gao L Z, Wang Z Q, Xu Z Q, Yang J N and Zhang W. 2010. A new evidence from zircon SHRIMP U_Pb dating of the Neoproterozoic diamictite in Quruqtagh area, Tarim Basin, Xinjiang, China[J]. Geological Bulletin of China, 29(2_3): 205_213(in Chinese with English abstract).
Gao L Z, Guo X P, Ding X Z, Zong W M, Gao Z J, Zhang C H and Wang Z Q. 2013. Nanhuan glaciation event and its stratigraphic correlation in Tarim Plate, China[J]. Acta Geoscientica Sinica, 34(1): 39_57 (in Chinese with English abstract).
Gao Z J and Zhu C C. 1984. The Xinjiang precambrian geology[M]. Urumqi: Xinjiang People Press.1_151(in Chinese).
Gao Z J, Wang W Y, Peng C W, Li Y A and Xiao B. 1985. Xinjiang Sinian system (one summary of the Xinjiang formation)[M]. Urumqi: Xinjiang People's Press. 1_173(in Chinese).
Gao Z J. 1993. North of Xinjiang precambrian[M]. Beijing: Geological Publishing House. 1_171(in Chinese).
Gao Z J and Chen K Q. 2003. The Nanhua system of Xinjiang and some geological issues of Nanhua system in China[J]. Geological Survey and Research, 26 (1): 8_13(in Chinese with English abstract).
Goodwin A M. 1973. Archean iron_formations and tectonic basins of the Canadian Shield[J]. Econ. Geol., 68(7): 915_933.
Griffin W L, Belousova E A and Shee S R. 2004. Archean crustal evolution in the northern Yilgam Craton: U_Pb and Hf isotope evidence from detrital zircons[J]. Precambrian Research, 131(3_4): 231_282.
Gross G A. 1980. A classification of iron formations based on depositional environments[J]. Canadian Mineralogist, 18(2): 215_222.
Gross G A. 1983. Tectonic systems and the deposition of iron_formation[J]. Precambrian Research, 20(2_4): 171_187.
Halverson G P, Poitrasson F, Hoffman P F, Nedelec A, Montel J M and Kirby J. 2011. Fe isotope and trace element geochemistry of the Neoproterozoic syn_glacial rapitan iron formation[J]. Earth and Planetary Science Letters, 309(1_2): 100_112.
Hayes J M and Waldbauer J R. 2006. The carbon cycle and associated redox processes through time[J]. Philosophical Transactions of the Royal Society Biological Sciences, 361(1470): 931_950.
He J W, Zhu W B, Zheng B H, Wu H L, Ge R F and Luo M. 2015. Provenance of Sinian Sugetbrak sedimentary rocks in the Aksu area, NW Tarim: Evidence from detrital zircon geochronology[J]. Acta Geologica Sinica, 89(1): 149_162(in Chinese with English abstract).
Hoffman P F, Kaufman A J and Halverson G P. 1998. A Neoproterozoic snowball earth[J]. Science, 281(5381): 1342_1346.
Hoffman P F and Schrag D P. 2002. The snowball earth hypothesis: Testing the limits of global change[J]. Terra Nova, 14(3): 129_155.
Hoffman P F, Macdonald F A and Halverson G P. 2011. Chapter 5 chemical sediments associated with Neoproterozoic glaciation: Iron formation, cap carbonate, barite and phosphorite[J]. Geological Society London Memoirs, 36(1): 67_80.
Holland H D. 1973. The oceans: A possible source of iron in iron formation[J]. Econ. Geol., 68(7): 1169_1172.
Hu A Q, Wang Z G and Tu G Z. 1997. Geological evolution and metallogenic regularity of the northern Xinjiang[M]. Beijing: Science Press. 1_246(in Chinese).
Huston D L and Logan G A. 2004. Barite, BIFs and bugs: Evidence for the evolution of the Earth's early hydrosphere[J]. Earth and Planetary Science Letters, 220(1): 41_55.
Ilyin A V. 2009. Neoproterozoic banded iron formations[J]. Lithology and Mineral Resources, 44(1): 78_86.
Jackson S E, Pearson N J and Griffin W L. 2004. The application of laser ablation microprobe_inductively coupled plasma_mass spectrometry (LAM_ICP_MS) to in situ U_Pb zircon geochronology[J]. Chemical Geology, 211(3): 47_69.
James H L. 1983. Chapter 12 distribution of banded iron_formation in space and time[M]. Developments in Precambrian Geology. Elsevier B.V: 471_490.
Johnson C M, Beard B L, Klein C, Beukes N J and Roden E E. 2008. Iron isotopes constrain biologic and abiologic processes in banded iron formation genesis[J]. Geochimica et Cosmochimca Acta, 72(1): 151_169.
Keys J R. 1979. Saline discharge at the terminus of the Taylor Glacier[J]. Antarctic Journal of the US, 14(5): 82_85.
Klein C and Beukes N J. 1993. Sedimentology and geochemistry of the Late Proterozoic glaciogenic rapitan iron formation in Canada[J]. Econ. Geol., 88(3): 542_565.
Klein C and Ladeira E A. 2004. Geochemistry and mineralogy of Neoproterozoic banded iron_formations and some selected, siliceous manganese formations from the Urucum district, Mato Grosso Do Sul, Brazil[J]. Econ. Geol., 99 (6): 1233_1244.
Klein C. 2005. Some Precambrian banded iron_formations (BIFs) from around the world: Their age, geologic setting, mineralogy, metamorphism, geochemistry, and origin[J]. American Mineralogist, 90: 1473_1499.
Kump L R and Seyfried J W E. 2005. Hydrothermal Fe fluxes during the Precambrian: Effect of low oceanic sulfate concentrations and low hydrostatic pressure on the composition of black smokers[J]. Earth and Planetary Science Letters, 235(3): 654_662.
Le Heron D P, Craig J and Etienne J L. 2009. Ancient glaciations and hydrocarbon accumulations in North Africa and the Middle East[J]. Earth Science Reviews, 93(3_4): 47_76.
Lechte M A and Wallace M W. 2016. Subice shelf ironstone deposition during the Neoproterozoic Sturtian glaciation[J]. Geology, 44(11): 891_894.
Lei R X, Wu C Z and Li G R. 2016. Neoproterozoic banded iron formation in the Central Tianshan, NW China: The Shalong example[J]. Acta Geologica Sinica, 90(4): 1543_1544.
Li H M. 2012. Classification of sedimentary metamorphic iron ore[J]. Mineral Deposits, 31(Supp.): 117_118(in Chinese).
Li W Q, Beard B L and Johnson C M. 2015. Biologically recycled continental iron is a major component in banded iron formations[J]. Proceedings of the National Academy of Sciences of the United States of America, 112(27): 8193_8198.
Li Y H, Hou K J, Wan D F and Zhang Z J. 2012. A compare geochemistry study for Algoma_and Superior_type banded iron formations[J]. Acta Petrologica Sinica, 28(11): 3513_3519(in Chinese with English abstract).
Li Y H, Zhang Z J, Hou K J, Duan C, Wan D F and Hu G Y. 2014. The genesis of Gongchangling high_grade_iron ores, Anshan_Benxi area, Liaoning Province, NE China: Evidence from Fe_Si_O_S isotopes[J]. Acta Geologica Sinica, 88(12): 2351_2372(in Chinese with English abstract).
Li Z H, Zhu X K and Tang S H. 2008. Characters of Fe isotopes and rare earth elements of banded iron formations from Anshan_Benxi area:Implications for Fe source[J]. Acta Petrologica et Mineralogica, 27(4): 285_290(in Chinese with English abstract).
Li Z H, Zhu X K and Sun J. 2014. Geochemical characters of Banded Iron Formations from Xinyu and North China[J]. Acta Petrologica Sinica, 30(5): 1279_1291(in Chinese with English abstract).
Li Z X, Evans D A and Halverson G P. 2013. Neoproterozoic glaciations in a revised global palaeogeography from the breakup of Rodinia to the assembly of Gondwanaland[J]. Sedimentary Geology, 294(294): 219_232.
Liu Q Q, Li Y F, Luo Z Z, Xie K J and Huang Z L. 2014. Geochemical characteristics of Jingshansi iron deposit in Wuyang, Henan Province, and their geological significance[J]. Mineral Deposits, 33(4): 697_712(in Chinese with English abstract).
Lottermoser B G and Ashley P M. 2000. Geochemistry, petrology and origin of Neoproterozoic ironstones in the eastern part of the Adelaide Geosyncline South Australia[J]. Precambrian Research, 101(1): 49_67.
Lu S N. 1992. Geological evolution of Proterozoic in Kuruqtage, Xinjiang[C]. Chinese Academy of Geological Sciences, (26_27): 279_292(in Chinese with English abstract).
Ludwig K R. 2003. User's Manual for Isoplot 3.0: Geochronological toolkit for Microsoft excel[M]. Berkeley Geochronology Center Special Publication. 1p.
Lyons W B, Welch K A and Sharma P. 1998. Chlorine_36 in the waters of the McMurdo Dry Valley Lakes, southern Victoria Land, Antarctica: Revisited[J]. Geochimica et Cosmochimica Acta, 62(2): 185_191.
Manikyamba C. 1999. Reworking of BIF into GIF in the Sandur Schist Belt, India: Possible evidence of sea level changes in an Archaean Proto_ocean[J]. Geological Society of India, 53(4): 453_461.
Michard A and Albarede F. 1986. The REE contents of some hydrothermal fluids[J]. Chemical Geology, 55(1): 51_60.
Mikucki J A and Priscu J C. 2004a. Microbial life in Blood Falls: An ancient antarctic ecosystem[C]. Conference on Early Mars Geologic. Second Conference on Early Mars: Geologic, Hydrologic, and Climatic Evolution and the Implications for Life, 8023.
Mikucki J A, Foreman C M, Sattler B, Lyons W B and Priscu J C. 2004b. Geomicrobiology of Blood Falls: An iron_rich saline discharge at the terminus of the Taylor Glacier, Antarctica[J]. Aquatic Geochemistry, 10(3_4): 199_220.
Mikucki J A, Pearson A, Johnston D T, Turchyn A V, Farquhar J, Schrag D P and Lee P A. 2009. A contemporary microbially maintained subglacial ferrous “Ocean"[J]. Science, 324(5925): 397_400.
Norin E. 1935. Tertiary of the Tarim Basin[J]. Acta Geologica Sinica, Bull Bulletin of Geological Society of China, 14(3): 337_348.
Och L M and Shields_Zhou G A. 2012. The Neoproterozoic oxygenation event: Environmental perturbations and biogeochemical cycling[J]. Earth_Science Reviews, 110(1_4): 26_57.
Pourmand A, Dauphas N and Ireland T J. 2011. A novel extraction chromatography and MC_ICP_MS technique for rapid analysis of REE, Sc and Y: Revising CI_chondrite and Post_Archean Australian Shale (PAAS) abundances[J]. Chemical Geology, 291(1): 38_54.
Shen B F, Zhai A M, Yang C L and Cao X L. 2005.Temporal_spatial distribution and evolutional characters of precambrian iron deposits in China[J]. Geological Survey and Research, 28(4): 196_205(in Chinese with English abstract).
Shu L S, Deng X L, Zhu W B, Ma D S and Xiao W J. 2011. Precambrian tectonic evolution of the Tarim Block, NW China: New geochronological insights from the Quruqtagh domain[J]. Journal of Asian Earth Sciences, 42 (5): 774_790.
Sial A N, Campos M S, Gaucher C, Frei R, Ferreira V P, Nascimento R C, Pimentel M M and Pereira N S. 2015. Algoma_type Neoproterozoic BIFs and related marbles in the Serido Belt (NE Brazil): REE, C, O, Cr and Sr isotope evidence[J]. Journal of South American Earth Sciences, 61: 33_52.
Sims P K and James H L. 1984. Banded iron_formations of Late Proterozoic age in the Central eastern desert, Egypt: Geology and tectonic setting[J]. Econ. Geol., 79 (8):1777_1784.
Song B, Zhang Y H, Wan Y S and Jian P. 2002. Mount making and procedure of the SHRIMP dating[J].Geological Review, 48(s1): 27_30(in Chinese with English abstract).
Spence G H, Le Heron D P and Fairchild I J. 2016. Sedimentological perspectives on climatic, atmospheric and environmental change in the Neoproterozoic Era[J]. Sedimentology, 63(2): 253_306.
Stern R J, Mukherjee S K, Miller N R, Ali K and Johnson P R. 2013. ~750 Ma banded iron formation from the Arabian_Nubian Shield: Implications for understanding Neoproterozoic tectonics, volcanism, and climate change[J]. Precambrian Research, 239: 79_94.
Swanson_Hysell N L, Rose C V, Calmet C C, Halverson G P, Hurtgen M T and Maloof A C. 2010. Cryogenian glaciation and the onset of carbon_isotope decoupling[J]. Science, 328(5978): 608_611.
Tang J F, Fu H Q and Yu Z Q. 1987. Horizons, types and forming conditions of the Late Precambrian iron formation in southern China[J]. Mineral Deposit, 6(1): 1_10(in Chinese with English abstract).
Torsvik T H. 2003. The rodinia Jigsaw puzzle[J]. Science, 300(5624): 1379_1381.
Trendall A F. 2002. The significance of iron_formation in the Precambrian stratigraphic record[M]. International Association of Sedimentologists Special Publication, 33: 33_66.
Volkert R A, Monteverde D H, Friehauf K C, Gates A E, Dalton R F and Smith R C. 2010. Geochemistry and origin of Neoproterozoic ironstone deposits in the New Jersey Highlands and implications for the eastern Laurentian rifted margin in the North_Central Appalachians, USA[J]. The Geological Society of America Memoir, 13: 283_306.
Walker J C G. 1984. Suboxic diagenesis in banded iron formations[J]. Nature, 309(5966): 340_342.
Wang C L, Zhang L C , Liu L and Dai Y P. 2012. Research progress of Precambrian iron formations abroad and some problems deserving further discussion[J]. Mineral Deposits, 31(6): 1311_1325(in Chinese with English abstract).
Wang X D and Lü X B. 2014. Geochemical characteristics and indicative significance of BIF in Quruqtage of Xinjiang[J]. Mineral Deposits, 33(supp.): 457-458(in Chinese).
Wang Y X, Qian Z X and Zheng H H. 1996. REE geochemistry of lacustrine deposit on the fildes Peninsula[J]. Antarctic Research (Chinese Edition), 8(2): 35_41(in Chinese with English abstract).
Wu C Z, Santosh M, Chen Y J, Samson I M, Lei R X, Dong L H, Qu X and Gu L X. 2014. Geochronology and geochemistry of Early Mesoproterozoic meta_diabase sills from Quruqtagh in the northeastern Tarim Craton: Implications for breakup of the Columbia supercontinent[J]. Precambrian Research, 241(1): 29_43.
Xinjiang Bureau of Geology and Mineral Resources. 1995. Geological map 1∶50 000, Baohuagou sheet (K45E017024) and report of regional geological survey[R].
Xu B, Jian P, Zhang H F, Zou H B, Zhang L F and Liu D Y. 2005. U_Pb zircon geochronology and geochemistry of Neoproterozoic volcanic rocks in the Tarim Block of northwest China: Implications for the breakup of Rodinia supercontinent and Neoproterozoic glaciations[J]. Precambrian Research, 136(2): 107_123.
Xu B, Kou X W, Song B, Wei W and Wang Y. 2008. SHRIMP dating of the Upper Proterozoic volcanic rocks in the Tarim plate and constraints on the Neoproterozoic glaciation[J]. Acta Petrologica Sinica, 24(12): 2857_2862(in Chinese with English abstract).
Xu B, Xiao S H, Zou H B, Chen Y, Li Z X, Song B, Liu D Y, Zhou C M and Yuan X L. 2009. SHRIMP zircon U_Pb age constraints on Neoproterozoic Quruqtagh diamictites in NW China[J]. Precambrian Research, 168(3_4): 247_258.
Young G M. 2002. Stratigraphic and tectonic setting of Proterozoic glaciogenic rocks and banded iron formations: Relevance to the snowball earth debate[J]. Journal of African Earth Science, 35(4): 451_466.
Yu Z Q, Tang J F and Fu H Q. 1989. Geoligical features and origin of the Xinyu type iron deposits[J]. Mineral Deposits, 8(4): 1_10(in Chinese with English abstract).
Zhang J and Nozaki Y. 1996. Rare earth elements and yttrium in seawater: ICP_MS determination in the East Caroline, Coral Sea, and South Fiji Basin of the western South Pacific Ocean[J]. Geochimica et Cosmochimica Acta, 60(23): 4631_4644.
Zhang Y L, Wang Z Q, Yan Z, Wang T and Guo X Q. 2011. Provenance of Neoproterozoic rocks in Quruqtagh area, Xinjiang: Evidence from detrital zircon geochronology[J]. Acta Petrologica Sinica, 27(1): 121_132(in Chinese with English abstract).
附中文参考文献
曹晓峰, 吕新彪, 高翔, 刘月高, 朱江, 陈超, 王于建, 刘智, 张彬. 2012. 新疆库鲁克塔格前寒武纪地块岩浆热事件及构造演化[J]. 新疆地质, 30(4): 384_391.
陈志华, 黄元辉, 唐正, 王豪壮, 葛淑兰, 方习生, 韩喜彬, 王爱军, 武力, 朱志敏. 2015. 南极半岛东北部海域表层沉积物稀土元素特征及物源指示意义[J]. 海洋地质与第四纪地质, 35(3): 145_155.
冯本智, 周裕文, 迟仕福. 1995. 新疆库鲁克塔格地区前震旦纪地质与贵重、有色金属矿床[M]. 北京:地质出版社.
高林志, 王宗起, 许志琴, 杨经绥, 张维. 2010. 塔里木盆地库鲁克塔格地区新元古代冰碛岩锆石SHRIMP U_Pb年龄新证据[J]. 地质通报, 29(2_3): 205_213.
高林志, 郭宪璞, 丁孝忠, 宗文明, 高振家, 张传恒,王自强. 2013. 中国塔里木板块南华纪成冰事件及其地层对比[J]. 地球学报,34(1): 39_57.
高振家, 朱诚顺, 彭昌文, 李永安, 章森桂, 缪长泉. 1984. 新疆前寒武纪地质[M]. 乌鲁木齐: 新疆人民出版社. 1_151.
高振家, 王务严, 彭昌文, 李永安, 肖兵. 1985. 新疆震旦系 (新疆地层总结之一) [M]. 乌鲁木齐: 新疆人民出版社. 1_173.
高振家. 1993. 新疆北部前寒武系[M]. 北京: 地质出版社. 1_171.
高振家, 陈克强. 2003. 新疆的南华系及我国南华系的几个地质问题——纪念恩师王曰伦先生诞辰一百周年[J]. 地质调查与研究, 26(1): 8_13.
何景文, 朱文斌, 郑碧海, 吴海林, 葛荣峰, 罗梦. 2015. 塔里木西北缘阿克苏地区震旦系苏盖特布拉克组沉积物源分析:碎屑锆石年代学证据[J]. 地质学报, 89(1):149_162.
胡霭琴, 王中刚, 涂光炽. 1997. 新疆北部地质演化及成岩成矿规律[M]. 北京: 科学出版社. 1_246.
李厚民. 2012. 沉积变质铁矿的分类[J]. 矿床地质, 31(增刊): 117_118.
李延河, 侯可军, 万德芳, 张增杰. 2012. Algoma型和Superior型硅铁建造地球化学对比研究[J]. 岩石学报,28(11):3513_3519.
李延河, 张增杰, 侯可军, 段超, 万德芳, 胡古月. 2014. 辽宁鞍本地区沉积变质型富铁矿的成因: Fe、Si、O、S同位素证据[J]. 地质学报,88(12): 2351_2371.
李志红, 朱祥坤, 唐索寒. 2008. 鞍山_本溪地区条带状铁建造的铁同位素与稀土元素特征及其对成矿物质来源的指示[J]. 岩石矿物学杂志, 27(4): 285_290.
李志红, 朱祥坤, 孙剑. 2014. 江西新余铁矿的地球化学特征及其与华北BIFs铁矿的对比[J]. 岩石学报,30(5):1279_1291.
刘清泉, 李永峰, 罗正传, 谢克家, 黄自力. 2014. 河南舞阳经山寺铁矿床地球化学特征及其地质意义[J]. 矿床地质,33(4): 697_712.
陆松年. 1992. 新疆库鲁克塔格元古宙地质演化[C]. 中国地质科学院天津地质矿产研究所文集, (26_27): 279_292.
沈保丰, 翟安民, 杨春亮, 曹秀兰. 2005. 中国前寒武纪铁矿床时空分布和演化特征[J]. 地质调查与研究, 28(4):196_205.
宋彪, 张玉海, 万渝生, 简平. 2002. 锆石SHRIMP样品靶制作、年龄测定及有关现象讨论[J]. 地质论评, 48(s1): 27_30.
汤家富, 符鹤琴, 余志庆. 1987. 华南晚前寒武纪硅铁建造的层位、类型与形成条件[J]. 矿床地质, 31(6): 1311_1325.
王长乐, 张连昌, 刘利, 代堰锫. 2012. 国外前寒武纪铁建造的研究进展与有待深入探讨的问题[J]. 矿床地质, 6(1): 1_10.
王祥东, 吕新彪. 2014. 新疆库鲁克塔格地区BIF铁矿地球化学特征及其指示意义[J]. 矿床地质, 33(增刊): 457_458.
王一先, 钱志鑫, 郑洪汉. 1996. 南极菲尔德斯半岛湖泊沉积物的稀土地球化学[J]. 南极研究(中文版), 8(2): 35_41.
新疆地质矿产局. 1995. 中华人民共和国1∶50 000地质图保华沟幅(K45E017024)及区域地质报告[R].
徐备, 寇晓威, 宋彪, 卫巍, 王宇. 2008. 塔里木板块上元古界火山岩SHRIMP定年及其对新元古代冰期时代的制约[J]. 岩石学报, 24(12): 2857_2862.
余志庆, 汤家富, 符鹤琴. 1989. “新余式"铁矿地质特征及其成因[J]. 矿床地质, 6(4): 1_10.
张英利, 王宗起, 闫臻, 王涛, 郭现轻. 2011. 库鲁克塔格地区新元古代沉积物源分析: 来自碎屑锆石年代学的证据[J]. 岩石学报, 27(1):121_132.
Oxidation and deposition of iron_rich saline water below glacier cap: Genesis
of Neoproterozoic Quruqsay iron deposit on northern margin of Tarim craton
Abstract
Banded Iron Formations (BIFs) reappeared during the Neoproterozoic, after about 1 billion years of absence in sedimentary records from the last deposition of Superior_type BIFs at 1.8 Ga. This type of iron formation is commonly associated with deposition of Neoproterozoic glacial facies which proceeded with the Cambrian radiation of metazoans, reflecting dramatic environmental change. A detailed understanding of mechanisms of Fe oxidation and accumulation during the Cryogenian period provides insights into evolution of the Earth as a system and biological innovations during this critical geological interval. In this paper the authors studied the hematite_rich Quruqsay iron deposit on the north margin of Tarim craton, which is associated with Neoproterozoic glaciation but was rarely reported in previous studies. Based on zircon U_Pb dating and regional stratigraphic correlation, the authors constrained the deposition age of the Quruqsay iron deposit between the early Qingbaikou period and the late Nanhua period in Neoproterozoic. Zircon age spectrum and petrographic features show that the clastic materials associated with hematite deposits were mainly from the Duduanshan Group, which was the upper part of the formation of Qingbaikou system. Major and trace element compositions indicate that hematite from the Quruqsay iron deposit was formed in an oxygen rich environment, and the ore_forming materials were mainly derived from continental crust, with only a small proportion of contribution from hydrothermal fluids or seawater. Geological and geochemical evidence suggests the close affinity between iron and continental glacier in the Quruqsay iron deposit, which is radically different from the Cryogenian Raptian_type BIFs, for which hydrothermal origin of Fe is commonly proposed. The authors argue that the iron source for the Quruqsay deposit originated from saline water below the glacier cap, which liberated Fe2+ from subglacial rocks during the progression of glaciers, and the subglacial Fe2+ fluids were oxidized at the edge of the glacier when it migrated into the ocean. Such mechanism of Fe2+ mobilization and oxidation has been observed from a modern glacier system at the Taylor Glacier in Antarctica, known as the famous “Blood Falls". The authors hold that the Quruqsay iron deposit is an example of the “Blood Falls"_type iron formation, which has a unique iron mineralization model sourced from surficial continental rocks, which is distinct from commonly proposed Archean BIF models and the Raptian BIF models.
Key words: geochemistry,Neoproterozoic, iron formation, glaciation, Quruqsay, Tarim
文章编号: 0258_7106 (2018) 01_0001_26
Doi: 10.16111/j.0258_7106.2018.01.001
* 本文得到国家自然科学基金(编号: U1603114)和中国地质调查局工作项目(编号: 1212011140056)联合资助
收稿日期 2016_12_06; 改回日期 2018_11_01。 秦思婷编辑。
中图分类号: P618.31
文献标志码:A